Criteria for a Recommended Standard: Occupational Exposure to Heat and Hot Environments.
Summary Statement
This National Institute for Occupational Safety and Health (NIOSH) criteria document extensively reviews the scientific data on heat stress and hot environments and has updated the Criteria for a Recommended Standard: Occupational Exposure to Hot Environments, published in 1986. While the goal of the document is to provide OSHA with the scientific basis and recommendations for standard-setting, the information provided is extensive and useful for industry, unions and health care providers. This revision includes additional information about the physiological changes that result from heat stress; updated information about risk factors and prevention of heat stroke and associated symptoms as well as updated Recommended Exposure Limits for acclimatized workers, and useful tables, references and appendices.
February 2016
Contents
ForewordExecutive Summary
Abbreviations
Glossary
Symbols
Acknowledgments
1 Recommendations for an Occupational Standard for Workers Exposed to Heat and Hot Environments
- 1.1 Workplace Limits and Surveillance
- 1.2 Medical Monitoring
- 1.2.1 General
- 1.2.2 Preplacement Medical Evaluations
- 1.2.3 Periodic Medical Evaluations
- 1.2.4 Emergency Medical Care
- 1.2.5 Informaiton to Be Provided to the Responsible Healthcare Provider.
- 1.2.6 Responsible Healthcare Provider's Written Report of Medical Findings
- 1.3 Surveillance of Heat-related Sentinel Health Events
- 1.4 Posting of Hazardous Areas
- 1.4.1 Dangerous Heat Stress Areas
- 1.4.2 Emergency Situations
- 1.4.3 Additional Requirements for Warning Signs
- 1.5 Protective Clothing and Equipment
- 1.6 Worker Information and Training
- 1.7 Control of Heat Stress
- 1.7.1 General Requirements
- 1.7.2 Engineering Controls
- 1.7.3 Work and Hygienic Practices
- 1.7.4 Heat Alert Program
- 1.8 Recordkeeping
3 Heat Balance and Heat Exchange 4 Biologic Effects of Heat 5 Measurement of Heat Stress
- 5.1 Environmental Factors
- 5.2 Prediction of Meteorological Factors from the National Weather Service Data
- 5.3 Metabolic Heat
- 6.1 Engineering Controls
- 6.2 Administrative Controls
- 6.3 Personal Protective Clothing and Auxiliary Body Cooling
- 7.1 Worker Participation
- 7.2 Program Oversight
- 7.3 Medical Monitoring Program Elements
- 7.4 Medical Surveillance- Periodic Evaluation of Data
- 7.5 Employer Actions
- 7.6 Considerations Regarding Reproduction
- 8.1 The NIOSH RALs and RELs
- 8.2 Estimation of Risks
- 8.3 Correlation between Exposure and Effects
- 8.4 Physiologic Monitoring of Heat Strain
- 8.5 Recommendations of U.S. Organizations and Agencies
- 8.5.1 The American Conference of Governmental Industrial Hygienists (ACGIH)
- 8.5.2 Occupational Safety and Health Administration (OSHA)
- 8.5.3 American Industrial Hygiene Association (AIHA)
- 8.5.4 The Armed Services
- 8.5.5 American College of Sports Medicine (ACSM)
- 8.5.6 Washington State Department of Labor and Industries
- 8.5.7 Mine Safety and Health Administration (MSHA)
- 8.6 Intermational and Foreign Standards and Recommendations
- 9.1 Direct Indices
- 9.2 Rational Indices
- 9.3 Empirical Indices
- 9.3.1 The Effective Temperature (ET, CET, ET*, and P4SR)
- 9.3.2 The Wet Bulb Globe Temperature (WBGT)
- 9.3.3 Wet Globe Temperature (WGT)
- 9.3.4 Universal Thermal Climate Index (UTCI)
- 9.3.5 Psychometric Chart
- 9.4 Physiologic Monitoring
- 10.1 Exposure Times and Patterns
- 10.2 Deep Body Temperature
- 10.3 Electrolyte and Water Balance
- 10.4 Effects of Chronic Heat Exposure
- 10.5 Circadian Rhythm of Heat Tolerance
- 10.6 Heat Tolerance and Shift Work
- 10.7 Detection, Treatment, and Prevention of Heat-related Illnesses
- 10.8 The Effects of Global Climate Change
- 10.9 Heat Stress and Toxicology
- 10.10 Other Research Needs
Appendices
Foreword
When the U.S. Congress passed the Occupational Safety and Health Act of 1970 (Public Law 91-596), it established the National Institute for Occupational Safety and Health (NIOSH). Through the Act, Congress charged NIOSH with recommending occupational safety and health standards and describing exposure levels that are safe for various periods of employment, including but not limited to the exposures at which no worker will suffer diminished health, functional capacity, or life expectancy because of his or her work experience.
Criteria documents contain a critical review of the scientific and technical information about the prevalence of hazards, the existence of safety and health risks, and the adequacy of control methods. By means of criteria documents, NIOSH communicates these recommended standards to regulatory agencies, including the Occupational Safety and Health Administration (OSHA) and the Mine Safety and Health Administration (MSHA), health professionals in academic institutions, industry, organized labor, public interest groups, and others in the occupational safety and health community.
A criteria document, Criteria for a Recommended Standard: Occupational Exposure to Hot Environments, was prepared in 1972 and first revised in 1986. The revision presented here takes into account the large amount of new scientific information on working in heat and hot environments. This revision includes updated information on heat-related illnesses, risk factors affecting heat-related illness, physiological responses to heat, effects of clothing on heat exchange, and recommendations for control and prevention.
Occupational exposure to heat can result in injuries, disease, death, and reduced productivity. Workers may be at risk for heat stress when exposed to hot environments. Exposure to hot environments and extreme heat can result in illnesses, including heat stroke, heat exhaustion, heat syncope, heat cramps, and heat rashes, or death. Heat also increases the risk of workplace injuries, such as those caused by sweaty palms, fogged-up safety glasses, and dizziness.
NIOSH urges employers to use and disseminate this information to workers. NIOSH also requests that professional associations and labor organizations inform their members about the hazards of occupational exposure to heat and hot environments.
NIOSH appreciates the time and effort taken by the expert peer, stakeholder, and public reviewers, whose comments strengthened this document.
John Howard, MD
Director, National Institute for
Occupational Safety and Health
Centers for Disease Control and Prevention
Executive Summary
Occupational exposure to heat can result in injuries, disease, reduced productivity, and death. To address this hazard, the National Institute for Occupational Safety and Health (NIOSH) has evaluated the scientific data on heat stress and hot environments and has updated the Criteria for a Recommended Standard: Occupational Exposure to Hot Environments [NIOSH 1986a]. This document was last updated in 1986, and in recent years, including during the Deepwater Horizon oil spill response of 2010, questions were raised regarding the need for revision to reflect recent research and findings. In addition, there is evidence that heat stress is an increasing problem for many workers, particularly those located in densely populated areas closer to the equator where temperatures are expected to rise in relation to the changing climate [Lucas et al. 2014]. This revision includes additional information about the physiological changes that result from heat stress; updated information from relevant studies, such as those on caffeine use; evidence to redefine heat stroke and associated symptoms; and updated information on physiological monitoring and personal protective equipment and clothing that can be used to control heat stress.
Workers who are exposed to extreme heat or work in hot environments indoors or outdoors, or even those engaged in strenuous physical activities may be at risk for heat stress. Exposure to extreme heat can result in occupational illnesses caused by heat stress, including heat stroke, heat exhaustion, heat syncope, heat cramps, heat rashes, or death. Heat can also increase workers’ risk of injuries, as it may result in sweaty palms, fogged-up safety glasses, dizziness, and may reduce brain function responsible for reasoning ability, creating additional hazards. Other heat injuries, such as burns, may occur as a result of contact with hot surfaces, steam, or fire. Those at risk of heat stress include outdoor workers and workers in hot environments, such as fire fighters, bakery workers, farmers, construction workers, miners (particularly surface miners), boiler room workers, and factory workers.
In 2011, NIOSH published with the Occupational Safety and Health Administration (OSHA) a co-branded infosheet on heat illness. Through this combined effort, many recommendations were updated, including those on water consumption. In addition, factors that increase risk and symptoms of heat-related illnesses were more thoroughly defined. In 2013, NIOSH published “Preventing Heat-related Illness or Death of Outdoor Workers.” Outdoor workers are exposed to a great deal of exertional and environmental heat stress.
Chapters on basic knowledge of heat balance and heat exchange largely remain unchanged, although clothing insulation factors have been updated to reflect current International Organization for Standardization (ISO) recommendations. Additional information on the biological effects of heat has become available in recent studies, specifically increasing the understanding of the central nervous system, circulatory regulation, the sweating mechanism, water and electrolyte balance, and dietary factors. New knowledge has been established about risk factors that can increase a worker’s risk of heat-related illness. Those over the age of 60 are at additional risk for suffering from heat disorders [Kenny et al. 2010]. Additional studies have examined sex-related differences regarding sweat-induced electrolyte loss and whole-body sweat response, as well as how pregnancy affects heat stress tolerance [Meyer et al. 1992; Navy Environmental Health Center 2007; Gagnon and Kenny 2011]. As obesity and the increasingly overweight percentage of the population in the United States continue to increase, this is now a major health concern in workers. Heat disorders among the obese and overweight occur more frequently than in lean individuals [Henschel 1967; Chung and Pin 1996; Kenny et al. 2010]. Another factor affecting heat-related illness is use of drugs, including cocaine, alcohol, prescription drugs, and caffeine. Caffeine use has long been argued against, as it has a diuretic effect and may reduce fluid volume, leading to cardiovascular strain during heat exposure [Serafin 1996]. However, more recent studies have found that the effect of caffeine on heat tolerance may be much less than previously suspected [Roti et al. 2006; Armstrong et al. 2007a; Ely et al. 2011].
The definition of heat stroke has also changed in recent years. Heat stroke is now classified as either classic heat stroke or exertional heat stroke which is more common in workplace settings. Characteristics of the individual (e.g., age and health status), type of activity (e.g., sedentary versus strenuous exertion), and symptoms (e.g., sweating versus dry skin) vary between these two classifications [DOD 2003]. Re-education is needed in the workplace especially about symptoms. Many workers have incorrectly been taught that as long as they were still sweating they were not in danger of heat stroke.
Measurements of heat stress are largely unchanged since the last revision, although additional information has been added about bimetallic thermometers and the psychrometric chart. The latter is a useful graphic representation of the relationships among dry bulb temperature, wet bulb temperature, relative humidity, vapor pressure, and dew point temperature. Such charts are especially valuable for assessing the indoor thermal environment. In addition, many modern computers and mathematical models can be used to calculate heat stress indices, based on weather station data.
Heat stress can be reduced by modifying metabolic heat production or heat exchange by convection, radiation, or evaporation. In a controlled environment, these last three can be modified through engineering controls, including increasing ventilation, bringing in cooler outside air, reducing the hot temperature of a radiant heat source, shielding the worker, and using air conditioning equipment. Heat stress can also be administratively controlled through limiting the exposure time or temperature (e.g., work/rest schedules), reducing metabolic heat load, and enhancing heat tolerance (e.g., acclimatization). Although most healthy workers will be able to acclimatize over a period of time, some workers may be heat intolerant. Heat intolerance may be related to many factors; however, a heat tolerance test can be used to evaluate an individual’s tolerance, especially after an episode of heat exhaustion or exertional heat stroke [Moran et al. 2007]. Additional preventive strategies against heat stress include establishing a heat alert program and providing auxiliary body cooling and protective clothing (e.g., water-cooled garments, air-cooled garments, cooling vests, and wetted overgarments).
Employers should establish a medical monitoring program to prevent adverse outcomes and for early identification of signs that may be related to heat-related illness. This program should include preplacement and periodic medical evaluations, as well as a plan for monitoring workers on the job.
Health and safety training is important for employers to provide to workers and their supervisors before they begin working in a hot environment. This training should include information about recognizing symptoms of heat-related illness; proper hydration (e.g., drinking 1 cup [8 oz.] of water or other fluids every 15–20 minutes); care and use of heat-protective clothing and equipment; effects of various factors (e.g., drugs, alcohol, obesity, etc.) on heat tolerance; and importance of acclimatization, reporting symptoms, and giving or receiving appropriate first aid. Supervisors also should be provided with appropriate training about how to monitor weather reports and weather advisories.
The NIOSH Recommended Alert Limits (RALs) and Recommended Exposure Limits (RELs) were evaluated. It was determined that the current RALs for unacclimatized workers and RELs for acclimatized workers are still protective for most workers. No new data were identified to use as the basis for updated RALs and RELs. Most healthy workers exposed to environmental and metabolic heat below the appropriate NIOSH RALs or RELs will be protected from developing adverse health effects. The Wet Bulb Globe Temperature–based limits for acclimatized workers are similar to those of OSHA, the American Conference of Governmental Industrial Hygienists, the American Industrial Hygiene Association, and the ISO. In addition, the Universal Thermal Climate Index (UTCI), originally developed in 2009, is gaining acceptance as a means of determining environmental heat stress on workers [Blazejczyk et al. 2013].
During the 2014 peer review of the draft criteria document, concerns were expressed about the sufficiency of the scientific data to support the NIOSH ceiling limits for acclimatized and unacclimatized workers. In fact, many acclimatized workers live and work in temperatures above the ceiling limits without adverse health effects. Further consideration of the scientific data led to the decision to remove the ceiling limit recommendations from the document.
Although research has produced substantial new information since the previous revision of this document, the need for additional research continues. Two newer areas of research that will likely continue to grow are the effects of climate change on workers and how heat stress affects the toxic response to chemicals. It is likely but unclear to what extent global climate change will impact known heat-exposure hazards for workers, especially with regard to severity, prevalence, and distribution [Schulte and Chun 2009; Schulte et al. 2015]. Toxicological research has shown that heat exposure can affect the absorption of chemicals into the body. Most of what is known on this subject comes from animal studies, so a better understanding of the mechanisms and role of ambient environment with regard to human health is still needed [Gordon 2003; Gordon and Leon 2005]. With changes in the climate, the need for a better understanding will become increasingly important [Leon 2008].
In addition to the updated research, this criteria document includes more resources for worker and employer training. Information about the use of urine color charts, including a chart and additional information, is in Appendix B. The National Weather Service Heat Index is in Appendix C, along with the OSHA-modified corresponding worksite protective measures and associated risk levels.
NIOSH recommends that employers implement measures to protect the health of workers exposed to heat and hot environments. Employers need to ensure that unacclimatized and acclimatized workers are not exposed to combinations of metabolic and environmental heat greater than the applicable RALs/RELs (see Figures 8-1 and 8-2). Employers need to monitor environmental heat and determine the metabolic heat produced by workers (e.g., light, moderate, or heavy work). Additional modifications (e.g., worker health interventions, clothing, and personal protective equipment) may be necessary to protect workers from heat stress, on the basis of increases in risk. In hot conditions, medical screening and physiological monitoring are recommended. Employers, supervisors, and workers need to be trained on recognizing symptoms of heat-related illness; proper hydration; care and use of heat-protective clothing and equipment; effects of various risk factors affecting heat tolerance (e.g., drugs, alcohol, obesity, etc.); importance of acclimatization; importance of reporting symptoms; and appropriate first aid.
Employers should have an acclimatization plan for new and returning workers, because lack of acclimatization has been shown to be a major factor associated with worker heat-related illness and death. NIOSH recommends that employers provide the means for appropriate hydration and encourage their workers to hydrate themselves with potable water <15°C (59°F) made accessible near the work area. Workers in heat <2 hours and involved in moderate work activities should drink 1 cup (8 oz.) of water every 15–20 minutes, but during prolonged sweating lasting several hours, they should drink sports drinks containing balanced electrolytes. In addition, employers should implement a work/rest schedule and provide a cool area (e.g., air-conditioned or shaded) for workers to rest and recover. These elements are intended to protect the health of workers from heat stress in a variety of hot environments.
Abbreviations
Ab | Area, Body Surface |
ADu | Area, DuBois |
Ar | Area, Effective Radiating |
As | Area, Solar Radiation |
Aw | Area, Wetted |
Aw/SWADu × 100 | Wettedness, Percent of Skin |
ACGIH | American Conference of Governmental Industrial Hygienists |
ACSM | American College of Sports Medicine |
ADH | Antidiuretic Hormone |
AIHA | American Industrial Hygiene Association |
ATP | Adenosine Triphosphate |
BLS | Bureay of Labor Statistics |
BMI | Body Mass Index |
bpm | Beats Per Minute |
BUN | Blood Urea Nitrogen |
°C | Degrees Celsius |
C | Convection |
Cal/OSHA | California OSHA |
CDC | Centers for Disease Control and Prevention |
CET | Corrected Effective Temperature |
CK | Creatine Kinase |
CNS | Central Nervous System |
CPK | Creatine Phosphokinase |
CO | Cardiac Output |
CSF | Cerebrospinal Fluid |
DIC | Disseminated Intravascular Coagulation |
DOD | Department of Defense |
-E | Evaporative Heat Loss |
E | Evaporation |
ECG | Electrocardiogram |
Emax | Maximum Water Uptake |
Ereq | Amount of Sweat Evaporated to Maintain Body Heat Balance |
EHE | Extreme Heat Event |
EMS | Emergency Medical Services |
ET | Effective Temperature |
°F | Degrees Fahrenheit |
Fcl | Reduction Factor for Heat Exchange due to Clothing |
g | Gram |
GI | Gastrointestinal |
h | Hour |
H | Body Heat Content |
hc | Convective Heat Transfer Coefficient |
he | Evaporative Heat Transfer Coefficient |
hr | Radiative Heat Transfer Coefficient |
hr+c | Radiative + Convective Heat Transfer Coefficient |
HAP | Heat Alert Program |
HHE | Health Hazard Evaluation |
HR | Heart Rate |
HSI | Heat Stress Index |
HTT | Heat Tolerance Test |
Ia | Thermal Insulation, Still Air |
Icl | Thermal Insulation, Clothing |
Icl+Ia | Thermal Insulation, Effective |
im | Moisture Permeability Index of Clothing |
im/clo | Permeability Index-Insulation Ratio |
ISO | International Organization for Standardization |
K | Conduction |
kcal | Kilocalories |
kg | Kilogram |
kJ | Kilojoule |
L | Liter |
M | Metabolism |
Met | Unit of metabolism |
min | Minute |
mL | milliliter |
mmHg | Millimeters of Mercury |
MR | Metabolic Rate |
MRT | Mean Radiant Temperature |
ms-1 | Meters per Second |
MSHA | Mine Safety and Health Administration |
Na | Sodium |
NIOSH | National Institute for Occupational Safety and Health |
NFPA | National Fire Protection Association |
NOAA | National Oceanic and Atmospheric Administration |
NSAIDs | Nonsteroidal Anti-inflammatory Drugs |
OEL | Occupational Exposure Limit |
OSHA | Occupational Safety and Health Administration |
Pa | Pressure, atmospheric |
Psk | Pressure, Wetted Skin |
Psk,s | Pressure, Skin Temperature |
PCr | Creatine Phosphate |
PHEL | Physiological Heat Exposure Limit |
PPE | Personal Protective Clothing |
R | Radiation |
RAL | Recommended Alert Limit |
RAAS | Renin-Angiotensin-Aldosterone System |
REL | Recommended Exposure Limit |
RER | Respiratory Exchange Ratio |
RH | Relative Humidity |
S | Body Heat Storage |
SACHS | Standards Advisory Committee on Heat Stress |
SCBA | Self-contained Breathing Apparatus |
SR | Sweat Produced Per Unit Time |
SV | Stroke Volume |
SWA | Area of Skin Wet with Sweat |
¯ta | Temperature, Ambient |
¯tadb | Temperature, Adjusted Dry Bulb |
¯td | Temperature, Mean Body |
tcr | Temperature, Core body |
tdp | Temperature, Dew-point |
tg | Temperature, Globe |
tnwb | Temperature, Natural Wet Bulb |
to | Temperature, Operative |
tor | Temperature, Oral |
tr | Temperature, Radiant |
¯tr | Temperature, Mean Radiant |
tre | Temperature, Rectal |
tsk | Temperature, Skin |
¯tsk | Temperature, Mean Skin |
tty | Temperature, Tympanic |
twb | Temperature, Psychrometric Wet Bulb |
TLV® | Threshold Limit Value |
TWA | Time Weighted Average |
ULPZ | Upper Limit of the Prescriptive Zone |
UTCI | Universal Thermal Climate Index |
Va | Air Velocity |
·VE | Minute Ventilation |
·VO2max | Maximum Oxygen Consumption |
w | Wettedness, Skin |
W | Work |
WBGT | Wet Bulb Globe Temperature |
WGT | Wet Globe Temperature |
WHO | World Health Organization |
Glossary
Acclimatization: The physiological changes that occur in response to a succession of days of exposure to environmental heat stress and reduce the strain caused by the heat stress of the environment; and enable a person to work with greater effectiveness and with less chance of heat injury.
Area, DuBois (ADu): Total nude body surface area in square meters (m2), calculated from the DuBois formula, and based on body weight (kg) and height (m).
Area, Effective Radiating (Ar): Surface area of the body in square meters (m2) that exchanges radiant energy with a radiant source.
Area, Solar Radiation (As): Surface area of the body in square meters (m2) that is projected normal to the sun.
Area, Wetted (Aw): Square meters (m2) of skin area covered by sweat.
Body Heat Balance: Steady-state equilibrium between body heat production and heat loss to the environment.
Body Heat Balance Equation: Mathematical expression of relation between heat gain and heat loss, expressed as S = (M – W) ± C ± R ± K – E
Body Heat Storage (S): The change in heat content (either + or −) of the body.
Circadian Rhythm: Synchronized, rhythmic biological phenomena that occur on approximately a 24-hour cycle.
clo: A unit expression of the insulation value of clothing, 1 clo = 5.55 kcal·m2·h-1·°C-1. A clo of 1 is equal to the insulation required to keep a sedentary person comfortable at 21°C (~70°F). It is also sometimes expressed as 1 clo = 0.155 m2·°C·W-1.
Conductive Heat Transfer (K): The net heat exchange involving the direct transfer of heat via direct contact between two mediums (solid, liquid, or gas) that have a temperature differential.
Conductive Heat Transfer Coefficient (hk): The rate of heat transfer between two mediums (solid, liquid, or gas) that have a temperature differential, expressed as W·m-2·°C-1.
Convective Heat Transfer (C): The net heat exchange by convection between an individual and the environment.
Convective Heat Transfer Coefficient (hc): The rate of heat transfer between the body surface and the ambient air per square meters (m2) of skin surface, expressed as W·m-2·°C-1.
Evaporative Heat Loss (-E): Body heat loss by evaporation of water (sweat) from the skin, expressed as kcal or W.
Evaporative Heat Transfer (E): Rate of heat loss by evaporation of water from the skin or gain from condensation of water on the skin, expressed as kcal·h-1, W·m-2, or W.
Evaporative Heat Transfer Coefficient (he): The rate of heat exchange by evaporation between the body surface and the ambient air, as a function of the vapor pressure difference between the two and air velocity.
Heat Capacity: Mass multiplied by specific heat of a body.
Heat Content of Body: The product of mean body temperature (tb) and body heat capacity (body mass × tissue specific heat), with the latter being constant for any given body composition.
Heat Cramp: A heat-related illness characterized by spastic contractions of the voluntary muscles (mainly arms, hands, legs, and feet), usually associated with restricted salt intake and profuse sweating without significant body dehydration.
Heat Exhaustion: A heat-related illness characterized by elevation of core body temperature above 38°C (100.4°F) and abnormal performance of one or more organ systems, without injury to the central nervous system. Heat exhaustion may signal impending heat stroke.
Heat Strain: The physiological response to the heat load (external or internal) experienced by a person, in which the body attempts to increase heat loss to the environment in order to maintain a stable body temperature.
Heat Stress: The net heat load to which a worker is exposed from the combined contributions of metabolic heat, environmental factors, and clothing worn which results in an increase in heat storage in the body.
Heat Stroke: An acute medical emergency caused by exposure to heat from an excessive rise in body temperature [above 41.1°C (106°F] and failure of the temperature-regulating mechanism. Injury occurs to the central nervous system characterized by a sudden and sustained loss of consciousness preceded by vertigo, nausea, headache, cerebral dysfunction, bizarre behavior, and excessive body temperature.
Heat Syncope: Collapse and/or loss of consciousness during heat exposure without an increase in body temperature or cessation of sweating, similar to vasovagal fainting except that it is heat induced.
Heat Tolerance: The physiological ability to endure heat and regulate body temperature at an average or better rate than others, often affected by the individual’s level of acclimatization and physical conditioning.
Humidity, Relative (RH): The ratio of the water vapor present in the ambient air to the water vapor present in saturated air at the same temperature and pressure.
Hyperpyrexia: A body core temperature exceeding 40°C (104°F).
Hyperthermia: A condition where the core temperature of an individual is higher than 37.2°C (99°F). Hyperthermia can be classified as mild (37.2–38.5°C; 99–101.3°F), moderate (i.e., heat exhaustion [38.5–39.5°C; 101.3–103.1°F]), profound (>39.5°C; 103.1°F), or profound clinical hyperthermia (i.e., heat stroke [>40.5°C; 104.9°F]), and death can occur without treatment (>45°C; 113°F).
Maximum Oxygen Consumption (VO2 max): The maximum amount of oxygen that can be used by the body.
Metabolic Rate (MR): Amount of chemical energy transferred into free energy per unit time.
Metabolism (M): Transformation of chemical energy into free energy that is used to perform work and produce heat.
Prescriptive Zone: The range of environmental temperatures where exercise at a given intensity results in thermal equilibrium, i.e., no change in core body temperature.
Pressure, Atmospheric (Pa): Pressure exerted by the weight of the air, which averages 760 mmHg at sea level and decreases with altitude.
Pressure, Water Vapor (Pa): The pressure exerted by the water vapor in the air.
Qualified Health Care Professional: An individual qualified by education, training, and licensure/regulation and/or facility privileges (when applicable) who performs a professional service within his or her scope of practice in an allied health care discipline, and independently reports that professional service.
Radiant Heat Exchange (R): The net rate of heat exchange by radiation between two radiant surfaces of different temperatures.
Radiative Heat Transfer Coefficient (hr): Rate of heat transfer between two black surfaces per unit temperature difference, expressed as W·m-2·°C-1
Recommended Alert Limit (RAL): The NIOSH-recommended heat stress alert limits for unacclimatized workers.
Recommended Exposure Limit (REL): The NIOSH-recommended heat stress exposure limits for acclimatized workers.
Rhabdomyolysis: A medical condition associated with heat stress and prolonged physical exertion, resulting in the rapid breakdown of muscle and the rupture and necrosis of the affected muscles.
Standard Man: A representative human with a body weight of 70 kg (154 lb) and a body surface area of 1.8 m2 (19.4 ft2).
Sweating, Thermal: Response of the sweat glands to thermal stimuli.
Temperature, Adjusted Dry Bulb (tadb): The dry bulb temperature is the temperature of the air measured by a thermometer that is shielded from direct radiation and convection.
Temperature, Ambient (ta): The temperature of the air surrounding a body. Also called air temperature or dry bulb temperature.
Temperature, Ambient, Mean (¯ta): The mean value of several dry bulb temperature readings taken at various locations or at various times.
Temperature, Core Body (tcr): Temperature of the tissues and organs of the body. Also called Core Temperature.
Temperature, Dew-point (tdp): The temperature at which the water vapor in the air first starts to condense.
Temperature, Effective (ET): Index for estimating the effect of temperature, humidity, and air movement on the subjective sensation of warmth.
Temperature, Globe (tg): The temperature inside a blackened, hollow, thin copper globe measured by a thermometer whose sensing element is in the center of the sphere.
Temperature, Mean Body (¯tb): The mean value of temperature at several sites within the body and on the skin surface. It can be approximated from skin and core temperatures.
Temperature, Mean Radiant (¯tr): The mean surface temperature of the material and objects surrounding the individual.
Temperature, Mean Skin (¯tsk): The mean of temperatures taken at several locations on the skin, weighted for skin area.
Temperature, Natural Wet Bulb (tnwb): The wet bulb temperature under conditions of the prevailing air movement.
Temperature, Operative (to): The temperature of a uniform black enclosure within which an individual would exchange heat by convection and radiation at the same rate as in a nonuniform environment being evaluated.
Temperature, Oral (tor): Temperature measured by placing the sensing element under the tongue for 3 to 5 minutes.
Temperature, Psychrometric Wet Bulb (twb): The lowest temperature to which the ambient air can be cooled by evaporation of water from the wet temperature-sensing element with forced air movement.
Temperature, Radiant (tr): The point temperature of the surface of a material or object, calculated from the following: MRT = Tg + (1.8 Va0.5)(Tg - Ta), where MRT = Mean Radiant Temperature (°C), Tg = black globe temperature (°C), Ta = air temperature (°C), and Va = air velocity (m·s-1).
Temperature, Rectal (tre): Temperature measured 10 centimeters (cm) into the rectal canal.
Temperature, Skin (tsk): Temperature measured by placing the sensing element on the skin.
Temperature, Tympanic (tty): True tympanic temperature is measured by placing the sensing element directly onto the tympanic membrane and recording the temperature. Estimates of tympanic temperature are usually obtained by placing a device into the ear canal close to the tympanic membrane.
Temperature Regulation: The maintenance of body temperature within a restricted range under conditions of positive heat loads (environmental and metabolic) by physiologic and behavioral mechanisms.
Thermal Insulation, Clothing: The insulation value of a clothing ensemble.
Thermal Insulation, Effective: The insulation value of the clothing plus the still air layer.
Thermal Strain: The sum of physiologic responses of the individual to thermal stress.
Thermal Stress: The sum of the environmental and metabolic heat load imposed on the individual.
Total Heat Load: The total heat exposure of environmental plus metabolic heat.
Universal Thermal Climate Index (UTCI): This index takes into account the human thermo-physiological significance across the entire range of heat exchange and the applicability of whole-body calculations including local skin cooling; it is valid in all climates and seasons.
Wet Bulb Globe Temperature (WBGT): This is an environmental temperature arrived at by measuring dry air temperature, humidity, and radiant energy (i.e., usually direct sunlight being absorbed by clothing), used to calculate a thermal load on the person.
Wettedness, Skin (w): The amount of skin that is wet with sweat.
Wettedness, Percent of Skin: The percentage of the total body skin surface that is covered with sweat.
Work: Physical efforts performed using energy from the metabolic rate of the body.
Symbols
Symbol | Term | Units |
---|---|---|
Ab | Body surface area | m2 |
ADu | Body surface area, DuBois | m2 |
Ar | Skin area exposed to radiation | m2 |
Aw | Wetted area of skin | m2 |
C | Heat exchange by convection | W, W·m-2 |
CO | Cardiac output of blood per minute | L·min-1 |
Emax | Maximum water vapor uptake by the air at prevailing meteorological conditions | kg·h-1 |
Ereq | Amount of sweat that must be evaporated to maintain body heat balance | kg·h-1 |
Fcl | Reduction factor for loss of convective heat exchange due to clothing | dimensionless |
H | Body heat content | W |
hc | Convection heat transfer coefficient | W·m-2·°C-1;kcal·h-1·m-2·°C-1 |
he | Evaporative heat transfer coefficient | W·m-2·kPa-1 |
HR | Heart rate | bpm |
hr | Radiative heat transfer coefficient | W·m-2·°C-1;kcal·h-1·m-2·°C-1 |
hr+c | Radiative + convective heat transfer coefficient | W·m-2·°C-1;kcal·h-1·m-2·°C-1 |
Ia | Thermal insulation of still air layer | clo |
Icl | Thermal insulation of clothing layer | clo |
im | Moisture permeability index of clothing | dimensionless |
im/clo | Permeability index–insulation ratio | dimensionless |
K | Heat exchanged by conduction | W, W·m-2 |
kcal | Kilocalories | kcal·h-1 |
M | Metabolism | met |
Met | Unit of metabolism; | 1 met=50 kcal·m-2·h-1 |
mmHg | Pressure in millimeters of mercury | mmHg |
m·s-1 | Meters per second | m·sec-1 |
Pa | Water vapor pressure of ambient air | mmHg, kPa |
Psk | Water vapor pressure of wetted skin | mmHg, kPa |
Psk,s | Water vapor pressure at skin temperature | mmHg, kPa |
RH | Relative humidity | percent |
R | Radiant Heat exchange | W, W·m-2 |
S | Sweat produced | L |
SR | Sweat produced per unit time | g·min-1, g·h-1, kg·min-1, kg·h-1 |
SV | Stroke volume, or amount of blood pumped by the heart per beat | mL |
SWA | Area of skin wet with sweat | m2 |
%SWA | SWA/ADu × 100 = % of body surface wet with sweat | percent |
T | Absolute Temperature (t + 273) | °K |
Ta | Ambient air dry-bulb temperature | °C, °F |
tadb | Ambient dry-bulb temperature, adjusted for solar radiation | °C, °F |
tcr | Body core temperature | °C, °F |
Tdb | Dew point temperature | °C, °F |
Tg | Black globe temperature | °C, °F |
Tnwb | Natural wet-bulb temperature | °C, °F |
to | Operative temperature | °C, °F |
tr | Radiant temperature | °C, °F |
¯tr | Mean radiant temperature | °C, °F |
tre | Rectal temperature | °C, °F |
tsk | Skin temperature | °C, °F |
¯tsk | Mean skin temperature | °C, °F |
Tpwb | Psychrometric wet-bulb temperature | °C, °F |
¯tw | Mean radiant temperature of the surroundings | °C, °F |
twg | Wet globe temperature | °C, °F |
Va | Air velocity | m·s-1, fpm |
·VO2max | Maximum oxygen consumption | mL·kg-1·min-1 (a measure of aerobic fitness), or L·h-1 (a measure of total O2 consumed at peak or maximal effort) |
W | Work | kcal·h-1 |
µ | Mechanical efficiency of work | %, percent |
w | Skin wettedness | dimensionless |
σ | Stefan-Bolzmann constant | W·m-2·K-4 |
ε | Emittance coefficient | dimensionless |
Acknowledgments
For contributions to the technical content and review of this document, the authors acknowledge the following NIOSH contributors:
Education and Information Division
Paul Schulte, PhD (Director)
Thomas Lentz, PhD, MPH
Kathleen MacMahon, DVM, MS
Lauralynn Taylor McKernan, ScD, CIH
HeeKyoung Chun, DSc
Barbara Dames
Sherry Fendinger
Ralph Zumwalde
Division of Surveillance, Hazard Evaluations, and Field Studies
Gregory Burr, CIH
Judith Eisenberg, MD, MS
Melody Kawamoto, MD, MS
Mark Methner, PhD
Doug Trout, MD, MHS
Division of Safety Research
Larry Jackson, PhD
Emergency Preparedness and Response Office
Joseph Little, MSPH
Health Effects Laboratory Division
Dan Sharp, MD, PhD
Office of Mine Safety and Health Research
Christopher Pritchard, MS, PE
National Personal Protective Technology Laboratory
Christopher Coffey, PhD
Western States Division
Yvonne Boudreau, MD, MSPH
Office of the Director
John Decker, MS, RPh, CIH
John Piacentino, MD
Paul Middendorf, PhD, CIH
Nura Sadeghpour, MPH
Seleen Collins provided editorial support, and Vanessa Williams, Nikki Romero, and Gino Fazio contributed to the design and layout of this document.
Finally, the authors express special appreciation to the following individuals for serving as independent external reviewers and providing comments that contributed to the development of this document:
Thomas Bernard, PhD,
MS
College of Public Health
University of South Florida
Phillip Bishop, EdD, MSEd
Human Performance Laboratory
University of Alabama
Tord Kjellstrom, MD, PhD,
MEng
Environmental Health Consultant, Professor
Health and Environment International Trust
Mapua, New Zealand
John Muller, MD, MPH, FACOEM
Occupational and Environmental Medicine
Navy Marine Corps Public Health Center
Suzanne Schneider, PhD
Department of Health Exercise and Sports Sciences
University of New Mexico
Rosemary Sokas, MD, MOH
Professor and Chair
Department of Human Science
School of Nursing and Health Studies
Georgetown University
Comments on the external review draft of this document were also submitted to the NIOSH docket and regulations.gov by interested stakeholders and other members of the public. All comments were considered in preparing this final version of the document.
1| Recommendations for an Occupational Standard for Workers Exposed to Heat and Hot Environments
The National Institute for Occupational Safety and Health (NIOSH) recommends that worker exposure to heat stress in the workplace be controlled by complying with all sections of the recommended standard found in this document. Compliance with this recommended standard should prevent or greatly reduce the risk of adverse health effects to exposed workers. Heat-related occupational illnesses, injuries, and reduced productivity occur in situations in which the total heat load (environmental plus metabolic heat) exceeds the capacities of the body to maintain normal body functions. The reduction of adverse health effects can be accomplished by the proper application of engineering and work practice controls, worker training and acclimatization, measurements and assessment of heat stress, medical monitoring, and proper use of heat-protective clothing and personal protective equipment (PPE).
In this criteria document, total heat stress is considered to be the sum of the heat generated in the body (metabolic heat), plus the heat gained from the environment (environmental heat), minus the heat lost from the body to the environment. Environmental and/or metabolic heat stress results in physiological responses (heat strain) to promote the transfer of heat from the body back to the environment to maintain core body temperature [Parsons 2003]. Many of the bodily responses to heat exposure are desirable and beneficial. However, at some level of heat stress, a worker’s compensatory mechanisms are no longer capable of maintaining body temperature at a level required for normal body functions. As a result, the risk of heat-related illnesses, disorders, and other hazards increases. The level of heat stress at which excessive heat strain will result depends on the heat tolerance capabilities of the worker. However, even though there is a wide range of heat tolerance between workers, each worker has an upper limit for heat stress, beyond which the resulting heat strain can cause the worker to become a heat casualty. In most workers, appropriate repeated exposure to elevated heat stress causes a series of physiologic adaptations called acclimatization, whereby the body becomes more efficient in coping with the heat stress. Such an acclimatized worker can tolerate a greater heat stress before a harmful level of heat strain occurs.
The occurrence of heat-related illnesses among a group of workers in a hot environment, or the recurrence of such illnesses in individual workers, represents “sentinel health events”, which indicate that heat control measures, medical screening, or environmental monitoring measures may not be adequate [Rutstein et al. 1983]. One occurrence of heat-related illness in a particular worker indicates the need for medical inquiry about appropriate workplace protections. The recommendations in this document are intended to provide limits of heat stress so that workers’ risks of incurring heat-related illnesses and disorders are reduced.
Almost all healthy workers who are not acclimatized to working in hot environments and who are exposed to combinations of environmental and metabolic heat less than the applicable NIOSH Recommended Alert Limits (RALs; Figure 8-1) should be able to tolerate the heat stress (i.e., the sum of metabolic heat plus environmental heat, minus the heat lost from the body to the environment) without a substantial increase in their risk of incurring acute adverse health effects. Almost all healthy workers who are heat-acclimatized to working in hot environments and who are exposed to combinations of environmental and metabolic heat less than the applicable NIOSH Recommended Exposure Limits (RELs; Figure 8-2) should be able to tolerate the heat stress without incurring adverse effects. The estimates of both environmental and metabolic heat are expressed as 1-hour time-weighted averages (TWAs), as described by the American Conference of Governmental Industrial Hygienists (ACGIH) [ACGIH 2014]. In this criteria document, when not otherwise qualified, the term “healthy workers” refers to those who are physically and medically fit and do not require additional protection, modifications in acclimatization procedures, or additional physiological monitoring beyond the normal recommendations for the amount of heat exposure.
The medical monitoring program should be designed and implemented to minimize the risk to workers’ health and safety from any heat hazards in the workplace (see Chapters 4, 5, and 6). The medical monitoring program should provide both preplacement medical evaluations for those persons who are candidates for hot jobs and periodic medical evaluations for those workers who are currently working in hot jobs.
1.1 Workplace Limits and Surveillance
1.1.1 Recommended Limits
Unacclimatized workers
Total heat exposure to workers should be controlled so that unprotected (i.e., those not wearing PPE that would provide protection against heat) healthy workers who are not acclimatized to working in hot environments are not exposed to combinations of metabolic and environmental heat greater than the applicable RALs, given in Figure 8-1.
Acclimatized workers
Total heat exposure to workers should be controlled so that unprotected healthy workers who are acclimatized to working in hot environments are not exposed to combinations of metabolic and environmental heat greater than the applicable RELs, given in Figure 8-2. For additional information on acclimatization, see 4.1.5 Acclimatization to Heat.
Effect of Clothing
The recommended limits given in Figures 8-1 and 8-2 are for healthy workers who are physically and medically fit for the level of activity required by their jobs and who are wearing the conventional one-layer work clothing ensemble consisting of not more than long-sleeved work shirts and trousers (or equivalent). The RAL and REL values given in Figures 8-1 and 8-2 may not provide adequate protection if workers wear clothing with lower air and vapor permeability or insulation values greater than those for the conventional one-layer work clothing ensemble. In addition, some workers at increased risk may need additional modifications to be protected from heat stress. A discussion of these modifications to the RALs and RELs is given in 3.3 Effects of Clothing on Heat Exchange.
1.1.2 Determination of Environmental Heat
Measurement methods
In most situations environmental heat exposures should be assessed by the Wet Bulb Globe Thermometer (WBGT) method or equivalent techniques, such as Effective Temperature (ET), Corrected Effective Temperature (CET), or Wet Globe Temperature (WGT), which are then converted to estimated WBGT values. When air- and vapor-impermeable protective clothing is worn, the dry bulb temperature (ta) or the adjusted dry bulb temperature (tadb) is a more appropriate measurement than the WBGT, because impermeable clothing does not transfer humid heat loss but only dry heat loss (e.g., radiation, convection, and conduction) [Åstrand et al. 2003]. These temperature readings may be used to determine the degree of heat stress the worker is experiencing in the work environment and allow a qualified safety and health professional to determine how to mitigate that heat stress to prevent heat injury.
Measurement requirements
Environmental heat measurements should be made at or as close as feasible to the work area where the worker is exposed and represents the environmental heat conditions at the worker’s position. When a worker is not continuously exposed in a single hot area but moves between two or more areas with differing levels of environmental heat or when the environmental heat substantially varies at the single hot area, the environmental heat exposures should be measured at each area and during each period of constant heat levels where employees are exposed. Hourly TWA WBGTs should be calculated for the combination of jobs (tasks), including all scheduled and unscheduled rest periods.
Modifications of work conditions
Environmental heat measurements should be made at least hourly, during the hottest portion of each work shift, during the hottest months of the year, and when a heat wave occurs or is predicted. If two such sequential measurements exceed the applicable RAL or REL, then work conditions should be modified by use of appropriate engineering controls, work practices, or other measures until two sequential measures are in compliance with the exposure limits of this recommended standard.
Initiation of measurements
A WBGT or individual environmental factors profile (e.g., air- and vapor-impermeable protective clothing, etc.) should be established for each hot work area, as a guide for determining when engineering controls and/or work practices or other control methods should be instituted. After the environmental profiles have been established, measurements should be made as described in this section, during the time of year and days when the profile indicates that total heat exposures above the applicable RALs or RELs may be reasonably anticipated or when a heat wave has been forecast by the nearest National Weather Service station or other competent weather forecasting service.
1.1.3 Determination of Metabolic Heat
The metabolic contribution to the heat load on the worker must be estimated or measured to ensure a safe working environment. A screening to estimate metabolic heat load should be calculated for each worker who is performing light, moderate, or heavy work. The metabolic heat rate should be determined in order to determine whether the total heat exposure exceeds the applicable RAL or REL.
Whenever the combination of measured environmental heat (WBGT) and screening estimated metabolic heat exceeds the applicable RAL or REL (Figures 8-1 and 8-2), the metabolic heat production should be measured using indirect calorimetry (see Chapter 5) or an equivalent method. Although performing indirect calorimetry in the field or on-site may not be feasible, indirect calorimetry can be performed on subjects performing at similar work levels in a laboratory setting. This information could provide an estimate of the metabolic heat production at that workload and thus support decisions regarding RAL or REL. Alternatively, the responsible individual (i.e., qualified safety and health professional) may refer to the Compendium of Physical Activities for information on metabolic responses to various types of work in order to determine RALs and RELs [Ainsworth et al. 2011]. For a short list of activities and the associated metabolic heat rate, see Example.
Metabolic heat rates should be expressed as kilocalories per hour ( kcal·h-1) or as watts (W) for a 1-hour TWA task basis that includes all activities engaged in during each period of analysis and all scheduled and nonscheduled rest periods (1 kcal·h-1 = 1.16 W).
If a moderate-workload task was performed by an acclimatized 70-kg (154-lb) worker for the entire 60 minutes of each hour, then the screening estimate for the 1-hour TWA metabolic heat would be about 300 kcal·h-1 (348.9 W). In Figure 8-2, a vertical line at 300 kcal·h-1 (348.9 W) intersects the 60 min·h-1 REL curve at a WBGT of 27.8°C (82°F). Then, if the measured WBGT exceeds 27.8°C (82°F), the worker’s metabolic heat could be measured by the indirect open-circuit method or an equivalent procedure.
If the 70-kg worker was unacclimatized, Figure 8-1 indicates that metabolic heat measurement of the worker would be required above a WBGT of 25°C (77°F).
1.1.4 Physiologic Monitoring
Physiologic monitoring may be used as an alternative to determining the required estimates and measurements described in the preceding parts of this section. The total heat stress shall be considered to exceed the applicable RAL or REL when the physiological functions exceed the values given in 9.4 Physiological Monitoring. Heart rate, core body temperature, and body water loss can be assessed as measures of physiologic response to heat. More advanced methods and new tools are also available for physiologic monitoring (see 8.4 Physiologic Monitoring of Heat Strain and 9.4 Physiologic Monitoring).
1.2 Medical Monitoring
1.2.1 General
- The employer should institute a medical monitoring program for all workers who are or may be exposed to heat stress above the RAL, whether they are acclimatized or not. A medical monitoring program is essential to assess and monitor workers’ health and physical well-being both prior to and while working in hot environments; to provide emergency medical care or other treatment as needed and gather medical information (e.g., identify changes in health status, identify training needs for prevention efforts). More information is available in Chapter 7.
- The employer should ensure that all medical evaluations and procedures are performed by or under the direction of the responsible healthcare provider (e.g., licensed physician or other licensed and/or credentialed healthcare professional).
- The employer should provide the required medical monitoring without cost to the workers, without loss of pay, and at a reasonable time and place.
1.2.2 Preplacement Medical Evaluations
For the purposes of the preplacement medical evaluation, all workers should be considered to be unacclimatized to hot environments. At a minimum, the preplacement medical evaluation of each prospective worker for a hot job should include the following elements:
- A comprehensive work and medical history. The medical history should include a comprehensive review of all body systems as would be standard for a preplacement physical examination, along with specific questions regarding previous episodes of diagnosed heat-related illness, rhabdomyolysis, and questions aimed at determining acclimatization to the new employment environment.
- A comprehensive physical examination should be conducted. At the discretion of the responsible healthcare provider, candidates who anticipate increased stress of physical activity of the job in a hot environment, those over 50 years of age or those younger than 50 years of age with underlying cardiac risk factors may need to have additional testing (e.g., electrocardiogram (ECG) with interpretation by a cardiologist).
- An assessment of the use of therapeutic drugs, over-the-counter medications, supplements, alcohol, or caffeine that may increase the risk of heat injury or illness (see Chapter 7).
- An assessment of obesity, defined as a body mass index (BMI) ≥ 30. Measure height and weight to calculate body mass index according to the following formula: BMI = weight (in pounds) × 703 / [height (in inches)]2
- An assessment of the worker’s ability to wear and use any protective clothing and equipment, especially respirators, that is or may be required to be worn or used.
- Other factors and examination details included in 7.3.1.1 Preplacement Physical Examination.
1.2.3 Periodic Medical Evaluations
Periodic medical evaluations should be made available at least annually to all workers who may be exposed at the worksite to heat stress exceeding the RAL. At minimum, the employer should provide the evaluations specified above. If circumstances warrant (e.g., an increase in job-related heat stress or changes in health status), the medical evaluation should be offered at more frequent intervals at the discretion of the responsible healthcare provider.
1.2.4 Emergency Medical Care
If the worker develops signs or symptoms of heat stroke or heat exhaustion, the employer should provide immediate emergency medical treatment (e.g., call 911 and cool down the worker). Other non-life-threatening heat-related illnesses may be treated with appropriate first aid procedures (see Table 4-3).
1.2.5 Information to Be Provided to the Responsible Healthcare Provider
The employer should provide the following information to the responsible healthcare provider performing or responsible for the medical monitoring program:
- A copy of this recommended standard.
- A description of the affected worker’s duties and activities (e.g., shift schedules, work locations) as they relate to the worker’s environmental and metabolic heat exposure.
- An estimate of the worker’s potential exposure to workplace heat (both environmental and metabolic), including any available workplace measurements or estimates.
- A description of any protective equipment or clothing the worker uses or may be required to use.
- Relevant information from previous medical evaluations of the affected worker that is not readily available to the responsible healthcare provider.
1.2.6 Responsible Healthcare Provider’s Written Report of Medical Findings
The employer should obtain a written opinion from the responsible healthcare provider, which should include the following elements:
- Occupationally pertinent results of the medical evaluation.
- A medical opinion as to whether the worker has any medical conditions that would increase the health risk of exposure to heat in the work environment.
- An estimate of the individual’s tolerance to withstand hot working conditions (see 6.2.3 Enhancing Tolerance to Heat and 6.2.5 Screening for Heat Intolerance).
- An opinion as to whether the worker can perform the work required by the job (i.e., physical fitness for the job).
- Recommendations for reducing the worker’s risk for heat-related illness, which may include use of cooling measures, accommodations or limitations related to work/rest schedules and/or workload, or reassignment to another job, as warranted.
- A statement that the worker has been informed by the responsible healthcare provider of the results of the medical evaluation and any medical conditions that require further explanation or treatment. The worker is cleared to work in the hot environment so long as no adverse health effects occur. Specific findings, test results, or diagnoses that have no bearing on the worker’s ability to work in heat or a hot environment should not be included in the report to the employer. Safeguards to protect the confidentiality of the worker’s medical records should be enforced in accordance with all applicable federal and state privacy regulations and guidelines.
1.3 Surveillance of Heat-related Sentinel Health Events
1.3.1 Definition
Surveillance of heat-related sentinel health events is defined as the systematic collection and analysis of data concerning the occurrence and distribution of adverse health effects in defined populations at risk for heat injury or illness.
1.3.2 Requirements
In order to evaluate and improve prevention and control measures for heat-related effects (including the need for exposure assessment), the following should be obtained and analyzed for each workplace: (a) workplace modifications, (b) identification of highly susceptible workers, (c) data on the occurrence or recurrence in the same worker, (d) distribution in time, place, and person of heat-related adverse effects, and (e) environmental or physiologic measurements related to heat.
1.4 Posting of Hazardous Areas
1.4.1 Dangerous Heat Stress Areas
In work areas and at entrances to work areas or building enclosures where there is a reasonable likelihood of the combination(s) of environmental and metabolic heat exceeding the RAL/REL, readily visible warning signs should be posted. These signs should contain information on the required protective clothing or equipment, hazardous effects of heat stress on human health, and information on emergency measures for heat injury or illness. This information should be arranged as follows:
DANGEROUS HEAT STRESS AREA
HEAT STRESS–PROTECTIVE CLOTHING OR EQUIPMENT REQUIRED
HEAT STROKE OR OTHER HEAT-RELATED ILLNESS MAY OCCUR
1.4.2 Emergency Situations
In any area where there is a likelihood of heat stress emergency situations occurring, the warning signs required in this section should be supplemented with signs giving emergency and first aid instructions, as well as emergency contact information.
1.4.3 Additional Requirements for Warning Signs
All hazard warning signs should be printed in English and, where appropriate, in the predominant language of workers unable to read English. Workers unable to read the signs should be informed of the warning printed on the signs and the extent of the hazardous area(s). All warning signs should be kept clean and legible at all times.
1.5 Protective Clothing and Equipment
Engineering controls and safe work practices should be used to ensure that workers’ exposure to heat stress is maintained at or below the applicable RAL or REL specified. In addition, protective clothing and equipment (e.g., water-cooled garments, air-cooled garments, ice-packet vests, wetted overgarments, and heat-reflective aprons or suits) should be provided by the employer to the workers when the total heat stress exceeds the RAL or REL (see 6.3 Personal Protective Clothing and Auxiliary Body Clothing).
1.6 Worker Information and Training
1.6.1 Information Requirements
All new and current workers who work in areas where there is reasonable likelihood of heat injury or illness, and their supervisors, should be kept informed, through continuing education programs, of the following:
- Heat stress hazards.
- Predisposing factors.
- Relevant signs and symptoms of heat injury and illness.
- Potential health effects of excessive heat stress.
- General first aid as well as worksite-specific first aid procedures.
- Proper precautions for work in heat stress areas.
- Workers’ responsibilities for following proper work practices and control procedures to help protect the health and provide for the safety of themselves and their fellow workers, including instructions to immediately report to the supervisor the development of signs or symptoms of heat-related illnesses.
- The effects of therapeutic drugs, over-the-counter medications, alcohol, or caffeine that may increase the risk of heat injury or illness by reducing heat tolerance (see Chapter 7).
- The purposes for and descriptions of the environmental and medical monitoring programs and the advantages to the worker of participating in these surveillance programs.
- If necessary, proper use of protective clothing and equipment.
- Cultural attitude toward heat stress. A misperception may exist that someone can be “hardened” against the requirement for fluids when exposed to heat by deliberately becoming dehydrated before work on a regular basis. This misperception is dangerous and must be counteracted through educational efforts.
1.6.2 Training Programs
- The employer should institute a training program, conducted by persons qualified by experience or training in occupational safety and health, to ensure that all workers potentially exposed to heat stress and their supervisors have current knowledge of at least the information specified in this section. For each affected worker, the instructional program should include adequate verbal and/or written communication of the specified information. The employer should develop a written plan of the training program that includes a record of all instructional materials.
- The employer should inform all affected workers of the location of written training materials and should make these materials readily available, without cost to the affected workers.
1.6.3 Heat Stress Safety Data Sheet
- The information specified in this section should be recorded on a heat stress safety data sheet or on a form specified by the Occupational Safety and Health Administration (OSHA).
- In addition, the safety data sheet should contain:
- Emergency and first aid pro- cedures, including site-specific con- tact information.
- Notes to the responsible healthcare provider regarding classification, medical aspects, and prevention of heat injury and illness. These notes should include information on the category and clinical features of each injury and illness, predisposing factors, underlying physiologic disturbance, treatment, and prevention procedures.
- Emergency and first aid pro- cedures, including site-specific con- tact information.
1.7 Control of Heat Stress
1.7.1 General Requirements
- The employer should establish and implement a written program to reduce exposures to or below the applicable RAL or REL by means of engineering and work practice controls.
- Where engineering and work practice controls are not sufficient to reduce exposures to or below the applicable RAL or REL, they should be used to reduce exposures to the lowest level achievable by these controls and should be supplemented by the use of heat-protective clothing or equipment. In addition, a heat alert program should be implemented as specified in this section.
1.7.2 Engineering Controls
- The type and extent of engineering controls required to bring the environmental heat below the applicable RAL or REL can be calculated with the basic heat exchange formulae (see Chapters 4 and 5). When the environmental heat exceeds the applicable RAL or REL, the following control requirements should be used.
- When the air temperature exceeds the skin temperature, convective heat gain should be reduced by decreasing air temperature and/or decreasing the air velocity if it exceeds 1.5 meters per second (m·sec-1) (300 ft·min-1). When air temperature is lower than skin temperature, convective heat loss should be increased by increasing air velocity. The type, amount, and characteristics of clothing will influence heat exchange between the body and the environment.
- When the temperature of the surrounding solid objects exceeds skin temperature, radiative heat gain should be reduced by placing shielding or barriers that are radiant-reflecting or heat-absorbing between the heat source and the worker; by isolating the source of radiant heat; by increasing the distance to the heat source; or by modifying the hot process or operation.
- When necessary, evaporative heat loss should be increased by increasing air movement over the worker, by reducing the influx of moisture from steam leaks or from water on the workplace floors, or by reducing the water vapor content (humidity) of the air. The air and water vapor permeability of the clothing worn by the worker will influence the rate of heat exchange by evaporation.
1.7.3 Work and Hygienic Practices
- Work modifications and hygienic practices should be introduced to reduce both environmental and metabolic heat when engineering controls are not adequate or are not feasible. The most effective preventive work and hygienic practices for reducing heat stress include, but are not limited to the following:
- Limiting the time the worker spends each day in the hot environment by decreasing exposure time in the hot environment and/or increasing recovery time spent in a cool environment.
- Reducing the metabolic demands of the job by such procedures as mechanization, the use of special tools, or an increase in the number of workers per task.
- Increasing heat tolerance by instituting a heat acclimatization plan (see Table 4-1 Acclimatization in workers) and by increasing physical fitness.
- Training supervisors and workers to recognize early signs and symptoms of heat illnesses and to administer relevant first aid procedures.
- Implementing a buddy system in which workers are responsible for observing fellow workers for early signs and symptoms of heat intolerance, such as weakness, unsteady gait, irritability, disorientation, changes in skin color, or general malaise.
- Some situations may require workers to conduct self-monitoring, and a workgroup (i.e., workers, responsible healthcare provider, and safety manager) should be developed to make decisions on self-monitoring options and standard operating procedures.
- Providing adequate amounts of cool (i.e., less than 15°C [59°F]), potable water near the work area and encouraging all workers that have been in the heat for up to 2 hours and involved in moderate work activities to drink a cup of water (about 8 oz.) every 15 to 20 minutes. Individual, not communal, drinking cups should be provided. During prolonged sweating lasting more than 2 hours, workers should be provided with sports drinks that contain balanced electrolytes to replace those lost during sweating, as long as the concentration of electrolytes/carbohydrates does not exceed 8% by volume.
- Limiting the time the worker spends each day in the hot environment by decreasing exposure time in the hot environment and/or increasing recovery time spent in a cool environment.
1.7.4 Heat Alert Program
A written Heat Alert Program should be developed and implemented whenever the National Weather Service or other competent weather service forecasts that a heat wave is likely to occur the following day or days. A heat wave is indicated when the daily maximum temperature exceeds 35°C (95°F) or when the daily maximum temperature exceeds 32°C (90°F) and is 5°C (9°F) or more above the maximum reached on the preceding days. More details are described in 6.2.6 Heat Alert Program.
1.8 Recordkeeping
1.8.1 Environmental and Metabolic Heat Surveillance
- The employer should establish and maintain an accurate record of all measurements made to determine environmental and metabolic heat exposures to workers, as required in this recommended standard (see 1.1.2 Determination of Environmental Heat).
- Where the employer has determined that no metabolic heat measurements are required as specified in this recommended standard, the employer should maintain a record of the screening estimates relied upon to reach the determination (see 1.1.3 Determination of Metabolic Heat).
1.8.2 Medical Surveillance
The employer should establish and maintain an accurate record for each worker subject to medical monitoring, as specified in this recommended standard (see 1.2 Medical Monitoring).
1.8.3 Surveillance of Heat-related Sentinel Health Events
The employer should establish and maintain an accurate record of the data and analyses specified in this recommended standard (see 1.3 Surveillance of Heat-related Sentinel Health Events).
1.8.4 Heat-related Illness Surveillance
The employer should establish and maintain an accurate record of any heat illness or injury and the environmental and work conditions at the time of the illness or injury (see 7.4 Medical Surveillance—Periodic Evaluation of Data).
1.8.5 Heat Stress Tolerance Augmentation
The employer should establish and maintain an accurate record of all heat stress tolerance augmentation for workers by heat acclimatization procedures (see 4.1.5 Acclimatization to Heat) and/or physical fitness enhancement.
2| Introduction
Criteria documents are developed by the National Institute for Occupational Safety and Health (NIOSH) under the authority of section 20(a) (3) of the Occupational Safety and Health Act of 1970. Through the Act, Congress charged NIOSH with recommending occupational safety and health standards and describing exposure limits that are safe for various periods of employment. These limits include, but are not limited to, the exposures at which no worker will suffer diminished health, functional capacity, or life expectancy as a result of his or her work experience. By means of criteria documents, NIOSH communicates these recommended standards to regulatory agencies (including the Occupational Safety and Health Administration [OSHA] and the Mine Safety and Health Administration [MSHA]), health professionals in academic institutions, industry, organized labor, public interest groups, and others in the occupational safety and health community. Criteria documents contain a critical review of the scientific and technical information about the prevalence of hazards, the existence of safety and health risks, and the adequacy of control methods.
In 1972 NIOSH published the Criteria for a Recommended Standard: Occupational Exposure to Hot Environments [NIOSH 1972], and in 1986 it published a revised criteria document [NIOSH 1986a] and a companion pamphlet, “Working in Hot Environments, Revised 1986” [NIOSH 1986b]. These publications presented the NIOSH assessment of the potential safety and health hazards encountered in hot environments, regardless of the workplace, and recommended a standard to protect workers from those hazards.
Heat-related occupational illnesses and injuries occur in situations where the total heat load (environmental and metabolic) exceeds the capacities of the body to maintain homeostasis. In the 1986 documents, NIOSH recommended sliding scale limits based on environmental and metabolic heat loads. These recommendations were based on the relevant scientific data and industry experience at that time. This criteria document reflects the most recent NIOSH evaluation of the scientific literature and supersedes the previous NIOSH criteria documents. This document presents the updated criteria and methods for recognition, evaluation, and control of occupational heat stress by engineering and preventive work practices. It also addresses the recognition, treatment, and prevention of heat-related illnesses by providing guidance for medical supervision, hygienic practices, and training programs.
The recommended criteria were developed to ensure that adherence to them will (1) protect against the risk of heat-related illnesses and heat-related reduction in safety performance, (2) be achievable by techniques that are valid and reproducible and (3) be attainable by means of existing techniques. This recommended standard is also designed to prevent harmful effects from interactions between heat and toxic chemical and physical agents. The recommended environmental limits for various intensities of physical work, as indicated in Figures 8-1 and 8-2, are not upper tolerance limits for heat exposure for all workers but, rather, levels at which engineering controls, preventive work and hygienic practices, and administrative or other control procedures should be implemented in order to reduce the risk of heat-related illnesses, even in the least heat tolerant workers.
Despite efforts to prevent heat-related deaths and illnesses, they continue. A 2008 Centers for Disease Control and Prevention (CDC) report identified 423 worker deaths among U.S. agricultural industries (16% were crop workers) and nonagricultural industries during 1992–2006. The heat-related average annual death rate for the crop workers was 0.39 per 100,000 workers, compared with 0.02 for all U.S. civilian workers [Luginbuhl et al. 2008]. Even with heat-specific workplace regulations in place in California, heat-related illnesses and deaths still occur particularily in agricultural workers who are at additional risk (e.g., extreme conditions, lack of knowledge, poverty, seasonality, low level of education, and other vulnerabilities related to migratory status) [Stoecklin-Marois et al. 2013].
In 2010, 4,190 injury or illness cases arising from exposure to environmental heat among private industry and state and local government workers resulted in one or more days of lost work [Bureau of Labor Statistics 2011]. Eighty-six percent of the heat-affected workers were aged 16–54 years. In that same year, 40 workers died from exposure to environmental heat. The largest number of workers (18) died in the construction industry, followed by 6 deaths in natural resources (including agriculture) and mining, 6 deaths in professional and business services (including waste management and remediation), and 3 deaths in manufacturing. Eighty percent of the deaths occurred among workers 25–54 years of age. Because heat-related illnesses are often not recognized, and only illnesses involving days away from work are reported, the actual number of occupational heat-related illnesses and deaths is not known. Additionally, estimates of the number of workers exposed to heat are not available.
A study of OSHA citations issued between 2012 and 2013 revealed 20 cases of heat-related illness or death of workers [Arbury et al. 2014]. In most of these cases, employers had no program to prevent heat illness, or programs were deficient; and acclimatization was the program element most commonly missing and most clearly associated with worker death.
3| Heat Balance and Heat Exchange
An essential requirement for continued normal body function is that the deep body core temperature be maintained within the range of about 37°C (98.6°F) ± 1°C (1.8°F). Achieving this body temperature equilibrium requires a constant exchange of heat between the body and the environment. The rate and amount of the heat exchange are governed by the fundamental laws of thermodynamics of heat exchange between objects. The amount of heat that must be exchanged is a function of (1) the total heat produced by the body (metabolic heat), which typically range from about 1 kcal per kilogram (kg) of body weight per hour (1.16 W) at rest to 5 kcal·kg-1 body weight·h-1 (7 W) for moderately hard industrial work, and (2) the heat gained, if any, from the environment. The rate of heat exchange with the environment is a function of air temperature and humidity, skin temperature, air velocity, evaporation of sweat, radiant temperature, and type, amount, and characteristics of the clothing worn. Respiratory heat loss is generally of minor consequence except during hard work in very dry environments. The following is a simple version of the heat balance equation.
3.1 Heat Balance Equation
The basic heat balance equation is
S = (M−W) ± C ± R ± K−E
where
S = change in body heat content
(M−W) = total metabolism minus external work performed
C = convective heat exchange
R = radiative heat exchange
K = conductive heat exchange
E = evaporative heat loss
To solve the equation, measurement of metabolic heat production, air temperature, water-vapor pressure, wind velocity, and mean radiant temperature are required [Belding 1971; Ramsey 1975; Lind 1977; Grayson and Kuehn 1979; Goldman 1981; Nishi 1981; ISO 1982b; ACGIH 1985; DiBenedetto and Worobec 1985; Goldman 1985a,b; Horvath 1985; Havenith 1999; Malchaire et al. 2001].
3.2 Modes of Heat Exchange
The major modes of heat exchange between humans and the environment are convection, radiation, and evaporation. Conduction usually plays a minor role in workplace heat stress, other than for brief periods of body contact with hot tools, equipment, floors, or other items in the work environment, or for people working in water or in supine positions [Havenith 1999]. The equations for calculating heat exchange by convection, radiation, and evaporation are available in Standard International (SI) units, metric units, and English units. In SI units, heat exchange is in watts per square meter of body surface (W·m-2). The heat-exchange equations are available in metric and English units for both the seminude individual and the worker wearing a conventional long-sleeved work shirt and trousers. The values are in kcal·h-1 for the “standard man,” defined as one who weighs 70 kg (154 lb) and has a body surface area of 1.8 m2 (19.4 ft2). For the purpose of this discussion, only SI or metric units will be used. For workers who are smaller or larger than the standard man, appropriate correction factors must be applied [Belding 1971]. The equations utilizing the SI units for heat exchange by C, R, and E are presented in Appendix A.
3.2.1 Convection (C)
The rate of convective heat exchange between the skin of a person and the ambient air immediately surrounding the skin is a function of the difference in temperature between the ambient air (ta) and the mean weighted skin temperature (¯tsk) and the rate of air movement over the skin (Va). This relationship is stated algebraically for the “standard man” wearing the conventional one-layer work clothing ensemble as [Belding 1971]:
C = 7.0 Va0.6(ta − ¯tsk)
where
C = convective heat exchange, kcal·h-1
Va = air velocity in meters per second (m·sec-1)
ta = ambient air temperature °C
¯tsk = mean weighted skin temperature, usually assumed to be 35°C
When ta >35°C, there will be a gain in body heat from the ambient air by convection;
when ta <35°C, heat will be lost from the body to the ambient air by convection.
This basic convective heat-exchange equation in English units has been revised for the “standard man” wearing the conventional one-layer work clothing ensemble:
C = 0.65 Va0.6(ta − ¯tsk)
where
C = convective heat exchange in Btu·h-1
Va = air velocity in feet per minute (fpm)
ta = ambient air temperature °C (°F)
¯tsk = mean weighted skin temperature, usually assumed to be 35°C (95°F)
3.2.2 Radiation (R)
The radiative heat exchange is primarily a function of the temperature gradient between the mean radiant temperature of the surroundings (¯tw) and the mean weighted skin temperature (¯tsk). Radiant heat exchange is a function of the fourth power of the absolute temperature of the solid surroundings, less the skin temperature (Tw−Tsk)4, but an acceptable approximation for the conventional one-layer clothed individual is this [Belding 1971]:
R = 6.6 (¯tw − ¯tsk)
R = radiant heat exchange, kcal·h-1 or W·m-2
¯tw = mean radiant temperature of the solid surrounding surface, °C (°F)
¯tsk = mean weighted skin temperature
For the conventional one-layer clothed individual and English units, the equation becomes
R = 15.0 (¯tw − ¯tsk)
R = radiant heat exchange, Btu·h-1
¯tw = mean radiant temperature, °C (°F)
¯tsk = mean weighted skin temperature
3.2.3 Evaporation (E)
The evaporation of water (sweat) from the skin surface results in a heat loss from the body. The maximum evaporative capacity (and heat loss) is a function of air motion (Va) and the water vapor pressure difference between the ambient air (Pa) and the wetted skin at skin temperature (Psk). The equation for this relationship is for the conventional one-layer clothed worker [Belding 1971]:
E = 14Va0.6(Psk − Pa)
E = Evaporative heat loss, kcal·h-1
Va = air speed, m·s-1
Pa = water vapor pressure of ambient air, mmHg
Psk = vapor pressure of water on skin, assumed to be 42 mmHg (5.6 kPa) at a 35°C (95°F) skin temperature
This translates in English units for the conventional one-layer clothed worker to:
E = 2.4Va0.6(Psk− Pa)
E = Evaporative heat loss, Btu·h-1
Va = air velocity, f·min-1
Pa = water vapor pressure air, mmHg
Psk = water vapor pressure on the skin, assumed to be 42 mmHg (5.6 kPa) at a 95°F (35°C) skin temperature
3.2.4 Conduction (K)
This type of heat exchange involves the direct transfer of heat via direct contact between two mediums (solid, liquid, or gas) that have a temperature differential. Therefore, for the purposes of this document, rate of heat transfer depends on the temperature gradient between the skin surface and the surrounding surfaces (hot metal surface, ice vest against the skin) and the thermal qualities of the surfaces (e.g., water absorbs heat at a much greater rate than air or stone) [McArdle et al. 2010a]. Under most circumstances, conduction is small in comparison with radiation, convection, and evaporative losses. However, under special circumstances such as wearing ice vests or liquid circulating personal cooling systems, conduction becomes more important. The equation for this theoretical relationship is as follows:
hk =KA (T1 − T2) / l
hk = Heat exchange via conduction
K = thermal “conductivity” determined by the physical properties of the objects
A = area for conduction of heat, m2
l = distance between points at T1 and T2 for the conduction for heat, m
T1 = temperature of the warmer object, °C
T2 = temperature of the cooler object, °C
This equation could be rewritten to represent thermal conduction to or from skin, as follows:
hk = KA (Tskin − Tobject) / l
where
K = conductive heat exchange in kcal·h-1
3.3 Effects of Clothing on Heat Exchange
Clothing serves as a barrier between the skin and the environment to protect against normal environmental elements of heat, cold, moisture, and abrasion. Special clothing has been developed to add further protection against hazardous chemical, physical, and biologic agents. A clothing ensemble will, of necessity, alter the rate and amount of heat exchange between the skin and the ambient air by convection, conduction, radiation, and sweat evaporation. Therefore, to calculate heat exchange by each of these modes, it is necessary to apply correction factors that reflect the type, amount, and characteristics of the clothing being worn when the clothing differs substantially from the conventional one-layer work clothing (i.e., more than one layer and/or greater air and vapor impermeability). This clothing efficiency factor (Fcl) for dry heat exchange is nondimensional [Goldman 1978; McCullough et al. 1982; Vogt et al. 1982]. In general, the thicker and greater the air and vapor impermeability of the clothing barrier layer or layers, the more it interferes with convective, radiative, and evaporative heat exchange.
Corrections of the RAL and REL have been suggested to reflect the Fcl based on heat transfer calculation for a variety of environmental and metabolic heat loads and three clothing ensembles [Heat Stress Management Program for the Nuclear Power Industry: Interim Report 1986]. The conventional one-layer clothing ensemble was used as the basis for comparisons with the other clothing ensembles. When a two-layer clothing ensemble is worn, the RAL and REL should be lowered by 2°C (3.8°F). When a partially air and/or vapor impermeable ensemble or heat reflective or protective aprons, leggings, gauntlets, etc., are worn, the RAL and REL should be lowered 4°C (7.2°F). These suggested corrections of the RAL or REL are scientific judgments that have not been substantiated by controlled laboratory studies or long-term workplace experience.
In those workplaces where a vapor and air impermeable encapsulating ensemble must be worn, the WBGT is not the appropriate measurement of environmental heat stress. In these instances, the adjusted dry bulb air temperature (tadb) must be measured and used instead of the WBGT. Where the tadb exceeds approximately 20°C (68°F), physiologic monitoring (core body temperature and/or pulse rate) is required. The method used for measuring core body temperature and pulse rate will be determined by the circumstances, and proper advance planning (attaching the physiological monitoring system) must take place prior to donning of the impermeable PPE. This physiologic monitoring must be conducted on a time schedule based upon metabolic heat production and tadb. The suggested frequency of physiologic monitoring for moderate work varies from once every two hours at tadb of 24°C (75°F) to every 15 minutes for moderate work at tadb of 32°C (90°F) [NIOSH 1985].
3.3.1 Clothing Insulation and Non-evaporative Heat loss
Even without any clothing, a thin layer of still air (the boundary layer) is trapped next to the skin. This external still air film acts as a layer of insulation against heat exchange between the skin and the ambient environment. Typically, without body or air motion, this air layer (la) provides about 0.8 clo units of insulation. One clo unit of clothing insulation is defined as allowing 5.55 kcal·m2·h-1 of heat exchange by radiation and convection (HR+C) for each °C of temperature difference between the skin (at a mean skin temperature ¯tsk) and adjusted dry bulb temperature tadb = (ta+¯tr)/2. The mean skin temperature is the average of temperatures taken at several locations on the skin, weighted for skin area. The “weighting” system takes into consideration the amount of heat transfer over these various areas of skin. The areas of skin are typically chest, bicep, forearm, thigh, calf, and subscapular region. The weighting system used by Ramanathan [1964] is generally considered an accurate manner of determining mean skin temperature from these data. For the standard man with 1.8 m2 of surface area, the hourly heat exchange by radiation and convection (HR+C) can be estimated as
HR+C = (10/clo) (¯tsk − tadb)
Thus, the 0.8 clo still air layer limits the heat exchange by radiation and convection for the nude standard individual to about 12.5 kcal·h-1 (14.5 W) for each °C of difference between skin temperature and air temperature. A resting individual in still air producing 90 kcal·h-1 (104.7 W) of metabolic heat will lose about 11 kcal·h-1 (12.8 W or 12%) by respiration and about the same by evaporation of the body water diffusing through the skin. The worker will then have to sweat and lose heat by evaporation to eliminate some of the remaining 68 kcal·h-1 (79.1 W) of metabolic heat if the tadb is less than 5.5°C below tsk [Goldman 1981].
The still air layer is reduced by increasing air motion, reaching a minimal value of approximately 0.2 clo at air speeds above 4.5 m·sec-1 (890 f·min-1 or 10 mph). At this wind speed, 68 kcal·h-1 (79.1 W) can be eliminated from the skin without sweating at an air temperature only 1.4°C below skin temperature, that is, 68/(10/0.2) = 1.4°C.
Studies of clothing materials have led to the conclusion that the insulation provided by clothing is generally a linear function of its thickness. Differences in fibers or fabric weave have only very minor effects on insulation, unless these directly affect the thickness or the vapor or air permeability of the fabric. The function of the fibers is to maintain a given thickness of still air in the fabric and block heat exchange. The fibers are more conductive than insulating; increasing fiber density (as when trying to fit two socks into a boot that has been sized to fit properly with one sock) can actually reduce the insulation provided [Goldman 1981].
The typical value for clothing insulation is 1.57 clo·cm-1 of thickness (4 clo·inch-1). It is difficult to extend this generalization to very thin fabric layers or to garments like underwear, which may simply occupy an existing still air layer of not more than 0.5 cm thickness. These thin layers show little contribution to the intrinsic insulation of the clothing, unless there is (a) “pumping action” of the clothing layers by body motion (circulation of air through and between layers of clothing due to body movement); (b) compression of the clothing by pressure from other clothing, by objects in contact with the body, or by external wind; or (c) penetration of some of the wind (as a function of the air permeability of the outer covering fabric) into the trapped air layer [ASHRAE 1981b; Goldman 1981; McCullough et al. 1982]. Table 3-1 lists the intrinsic insulation contributed by adding each of the listed items of typical clothing ensembles. The total intrinsic insulation is not the sum of the individual items, but 80% of their total insulation value; this allows for an average loss of 20% of the sum of the individual items to account for the compression of one layer on the next. This average 20% reduction is a rough approximation, which is highly dependent on such factors as the nature of the fiber, the weave, the weight of the fabric, the use of foam or other nonfibrous layers, and the clothing fit and cut.
In summary, insulation is generally a function of the thickness of the clothing ensemble, and this, in turn, is usually a function of the number of clothing layers. Thus, each added layer of clothing, if not compressed, will increase the total insulation. That is why most two-layer protective clothing ensembles exhibit quite similar insulation characteristics and most three-layer systems are comparable, regardless of some rather major differences in fiber or fabric type [Goldman 1981].
Because clothing can significantly insulate the wearer from the external environment and trap body heat because of the insulation or low permeability, the thermal influence of the clothing has led to the development of clothing adjustment factors that can be used to determine the overall thermal stress of the wearer. An example of adjustments for the thermal properties of clothing appears in Table 3-2.
As can be seen from Table 3-2, adjustments to the total thermal stress can be made for a variety of clothing and PPE. This table is useful for determining the total heat stress that is imposed on the worker wearing these or similar types of clothing. More comprehensive lists are available in the literature or in ACGIH guidelines.
3.3.2 Clothing Permeability and Evaporative Heat Loss
Evaporative heat transfer through clothing tends to be affected linearly by the thickness of the ensemble. The moisture permeability index (im) is a dimensionless unit with a theoretical lower limit value of 0 for a vapor- and air-impermeable layer and an upper value of 1 if all the moisture that the ambient environment can take up (as a function of the ambient air vapor pressure and fabric permeability) can pass through the fabric. Since moisture vapor transfer is a diffusion process limited by the characteristic value for diffusion of moisture through still air, values of im approaching 1 should be found only with high wind and thin clothing. A typical im value for most clothing materials in still air is less than 0.5 (e.g., im will range from 0.45 to 0.48). Water repellent treatment, very tight weaves, and chemical protective impregnations can reduce the im value significantly. However, even impermeable layers seldom reduce the im value to zero, since an internal evaporation-condensation cycle is set up between the skin surface and the inner surface of the impermeable layer, which effectively transfers some heat from the skin to the vapor barrier. This shunting, by passing heat across the intervening insulation layers, can be reflected as an im value of about 0.08, even for a totally impermeable overgarment.
Very few fiber treatments have been found to improve the im index value of fabric layers; surfactants, which increase the number of free hydroxyl (OH) radicals on the fiber surface or which somehow improve wicking, appear to increase the im value of a fabric. However, the ultimate evaporative heat transferred from the skin through the clothing and external air layers to the environment is not simply a function of the im but a function of the permeability index–insulation ratio (im/clo). The maximum evaporative heat exchange with the environment can be estimated for the HR+C of a “standard man” with 1.8 m2 of surface area, as:
HEmax=10im/clo × 2.2(Psk−Pa)
The constant 2.2 is the Lewis number; Psk is the water vapor pressure of sweat (water) at skin temperature (tsk); and Pa is the water vapor pressure of the ambient air at air temperature, ta. Thus, the maximum evaporative transfer tends to be a linear, inverse function of insulation, if not further degraded by various protective treatments, which range from total impermeability to water repellent treatments [Goldman 1973, 1981, 1985a]. The Lewis number is derived from fraction of skin wettedness, latent heat, and evaporative heat transfer (he).
3.3.3 Physiologic Problems of Clothing
The percent of sweat-wetted skin surface area (w) needed to eliminate the required amount of heat from the body by evaporation can be estimated simply as the ratio of the required evaporative cooling (Ereq) and the maximum water vapor uptake capacity of the ambient air (Emax). A totally wetted skin = 100%.
w =Ereq/Emax
Having some sweat-wetted skin is not uncomfortable; in fact, some sweating during exercise in heat increases comfort. As the extent of skin wetted with sweat approaches 20%, the sensation of discomfort begins to be noted. Discomfort is marked and performance decrements can appear with between 20% and 40% wetting of the body surface; performance decrements become increasingly noted as w approaches 60%. Sweat begins to be wasted, dripping rather than evaporating at 70%; physiologic strain becomes marked between 60% and 80% w. Increases of w above 80% result in limited tolerance, even for physically fit, heat-acclimatized young workers. The above arguments indicate that any protective work clothing will pose some limitations on tolerance, since with Ia plus Iclo rarely below 2.5 clo, their im/clo ratios are rarely above 0.20 [Goldman 1985b].
The physiologic strain that arises with wearing clothing, heat transfer, and work can be estimated from equations that describe the competition for the blood pumped by the heart. The cardiac output (CO) is the stroke volume (SV) (or volume of blood pumped per beat) multiplied by heart rate (HR) in beats per minute (CO = SV × HR). The cardiac output increases essentially linearly with increasing work; the rate-limiting process for metabolism is the maximum rate of delivery of oxygen to the working muscle via the blood supply. It is expressed in liters per minute (L·min-1). In heat stress, this total blood supply must be divided between the working muscles and the skin where the heat exchange occurs. In this consideration, it is important to realize that not all of the CO is diverted to muscle and skin. Other organ systems must receive oxygenated blood during exercise as well, especially the brain, heart, and viscera [McArdle et al. 2010a].
SV rapidly reaches a constant value for a given intensity of work. Thus, the work intensity (i.e., the rate of oxygen delivered to the working muscles) is essentially indicated by HR; the individual worker’s maximum HR limits the ability to continue work. Conditions that impair the return of blood from the peripheral circulation to fill the heart between beats will affect work capacity. The maximum achievable HR is a function of age and can be roughly estimated by this relationship: 220 beats per minute (bpm) minus age in years [Hellon and Lind 1958; Drinkwater and Horvath 1979]. Given equivalent HR at rest (e.g., 60 bpm), a 20-year-old worker’s HR has the capacity to increase by 140 bpm, i.e., (220−20)−60, while a 60-year-old worker can increase his HR only 100 bpm, i.e., (220−60)−60. Because the demands of a specific task will be roughly the same for 20- and 60-year-old individuals who weigh the same and do the same amount of physical work, the decline in maximum achievable HR with age increases both the perceived and the actual relative physiologic strain of work on the older worker. Given the above, it is preferable to use a percent of the person’s HRmax rather than refer to a specific absolute HR as a target for work.
The ability to transfer the heat produced by muscle activity from the core of the body to the skin is also a function of the CO. Blood passing through core body tissues is warmed by heat from metabolism during rest and work. The basic requirement is that skin temperature (tsk) must be maintained at least 1°C (1.8°F) below deep body temperature (tre) if blood that reaches the skin is to be cooled before returning to the body core. The heat transferred to the skin is limited, ultimately, by the CO and by the extent to which tsk can be maintained below tre.
A worker’s tre is a function of metabolic heat production (M) (tre = 36.7 + 0.004M), as long as there are no restrictions on evaporative and convective heat loss by clothing, high ambient vapor pressures, or very low air motion; for example, at rest, if M = 105W, tre is about 37.1°C (98.8°F). Normally, under the same conditions of unlimited evaporation, skin temperatures are below tre by about 3.3°C + (0.006M); thus, at rest, when tre is 37°C, the corresponding tsk is about 33°C, that is, 37− (3.3 + 0.6). This 3° C–4°C difference between tre and tsk indicates that, at rest, each liter of blood flowing from the deep body to the skin can transfer approximately 4.6 watts or 4 kcal of heat to the skin. Since tre increases and tsk decreases from the evaporation of sweat with increasing M, it normally becomes easier to eliminate body heat with increasing work, since the difference between tre and tsk increases by about 1°C (1.8°F) per 100 watts (86 kcal) of increase in M (i.e., tre up 0.4°C (0.7°F), and tsk down 0.6°C (1.1°F) per 100 watts of M). Thus, at sustainable hard work (M = 500 watts or 430 kcal·h-1), each liter of blood flowing from core to skin can transfer 9 kcal (10.5 W) to the skin, which is 2.5 times that at rest [Goldman 1973, 1985a].
Work under a heat stress condition sets up a competition for CO, particularly as the blood vessels in the skin dilate to their maximum and less blood is returned to the central circulation. Gradually, less blood is available in the venous return to fully fill the heart between beats, causing the SV to decrease; therefore, HR must increase to maintain the same CO. For fit, young workers, the average work HR should be limited to about 110 bpm if an 8-hour work shift is to be completed; an average HR of 140 bpm should be maintained for no more than 4 hours, and an average HR of 160 bpm should be maintained for no more than 2 hours [Brouha 1960]. If the intensity of work results in a HR in excess of these values, then the intensity of work should be reduced. Thus, heat added to the demands of work rapidly results in problems, even in healthy, young workers. These problems are amplified if circulating blood volume is reduced as a result of inadequate water intake to replace sweat losses, which can average one liter an hour over an 8-hour work shift, or by vomiting, diarrhea, or diuresis.
The crisis point, heat exhaustion and collapse, is a manifestation of inadequate blood supply to the brain; this occurs when CO becomes inadequate because of insufficient return of blood from the periphery to fill the heart for each beat or because of inadequate time between beats to fill the heart as HR approaches its maximum.
Clothing interferes with heat loss from the skin, and skin temperature rises predictably with increased clothing. Because of the insulation-induced rise in tsk and the resultant limited ability to dissipate heat that has been transferred from the core to the skin, core temperature (tre) also rises when clothing is worn. Another type of interference with heat loss from the skin arises when sweat evaporation is required for body cooling (i.e., when M + HR + C > O) but is limited by high ambient water vapor pressure, low wind, or low clothing permeability index (im/clo).
As Ereq approaches Emax, skin temperature increases dramatically and deep body temperature begins to increase rapidly. Deep body temperatures above 38.0°C (100.4°F) are considered undesirable for an average worker. The risk of heat-exhaustion collapse is about 25% at a deep body temperature of 39.2°C (102.6°F), associated with a skin temperature of 38°C (100.4°F) (i.e., tsk converging toward tre and approaching the 1°C [1.8°F] limiting difference where one liter of blood can transfer only 1 or 2 kcal·h-1[1.16 W or 2.33 W] to the skin). At a similarly elevated tsk where tre is 39.5°C (103.1°F), there is an even greater risk of heat-exhaustion collapse, and as tre approaches 40°C (104°F), with elevated skin temperatures, most individuals are in imminent danger of heat-related illness. Finally, tre levels above 41°C (105.8°F) are associated with heat stroke, a life-threatening major medical emergency. The competition for CO is exacerbated by dehydration (limited SV), age (limited maximum HR), and reduced physical fitness (compromised CO). These work-limiting and potentially serious deep body temperatures are reached more rapidly when combinations of these three factors are involved.
As indicated in the above statements, maximum work output may be substantially degraded by almost any protective clothing worn during either heavy work in moderately cool environments or low work intensities in hot conditions, because of the clothing interfering with heat elimination. Heat stress is also likely to be increased with any two-layer protective ensembles or any effective single-layer vapor barrier system for protection against toxic products, unless some form of auxiliary cooling is provided [Goldman 1973, 1985a].
4|Biologic Effects of Heat
4.1 Physiologic Responses to Heat
4.1.1 The Central Nervous System
The central nervous system is responsible for the integrated organization of thermoregulation. The hypothalamus is thought to be the central nervous system’s primary seat of control. Historically, in general terms, the anterior hypothalamus has been considered to function as an integrator and “thermostat,” whereas the posterior hypothalamus provides a “set point” of the core or deep-body temperature and initiates the appropriate physiologic responses to keep the body temperature at that set point if the core temperature changes.
According to this model, the anterior hypothalamus receives the information from receptors sensitive to changes in temperature in the skin, muscle, stomach, other central nervous system tissues, and elsewhere. In addition, the anterior hypothalamus itself contains neurons that are responsive to changes in temperature of the arterial blood serving the region. The neurons responsible for the transmission of the temperature information use monoamines, among other neurotransmitters; this has been demonstrated in animals [Cooper et al. 1982]. These monoamine transmitters are important in the passage of appropriate information to the posterior hypothalamus. It is known that the set point in the posterior hypothalamus is regulated by ionic exchanges. However, the set-point hypothesis has generated considerable controversy [Greenleaf 1979]. The problem with the notion of a set point is that (1) a neuroanatomical region controlling the set point has never been identified and (2) the physiological responses to heat cannot be explained by the notion of a set point. At present, it appears that the hypothalamic region does integrate neural traffic from thermoreceptors and integrates a physiological response to an increase in temperature. However, the current data suggest that the hypothalamus controls temperature within a so-called inter-thermal threshold (range of temperatures around a mean with which no physiological response occurs). The physiological responses occur only when the temperature moves beyond the “thresholds” to elicit either sweating or thermogenesis and the appropriate vasomotor response (i.e., vasoconstriction or vasodilation) [Mekjavic and Eiken 2006]. The ratio of sodium to calcium ions is also important in thermoregulation. The sodium ion concentration in the blood and other tissues can be readily altered by exercise and by exposure to heat.
When a train of neural traffic is activated from the anterior to the posterior hypothalamus, it is reasonable to suppose that once a “hot” pathway is activated, it will inhibit the function of the “cold” pathway and vice versa. However, there is a multiplicity of neural inputs at all levels in the central nervous system, and many complicated neural “loops” undoubtedly exist.
Current research suggests that, instead of the historical notion of a set point, neural input into the hypothalamus is integrated into a response that can be described as “cross inhibitory.” In other words, when neural inputs from warm thermoreceptors in the skin are dominant, the integrated response results in an increase in sweating and cutaneous vasodilation, while simultaneously inhibiting thermogenesis and vice versa [Mekjavic and Eiken 2006]. Paradoxically, when appropriate, the core body temperature will increase and be regulated at a higher level in order to maintain an increase in heat loss, by maintaining a thermal gradient between the core body temperature and the skin temperature for the transfer of heat to the environment [Taylor et al. 2008]. Although this discussion focuses on the role of the anterior hypothalamus in heat stress and injury, there are many other factors that influence the anterior hypothalamus and the control of heat balance. These factors include thyroid and reproductive hormones, cerebrospinal fluid (CSF) ions and osmolality, glucose, and input from other brain regions related to arousal, circadian variation, and menstrual function [Kandel and Schwartz 2013].
A question that must be addressed is the difference between a physiologically raised body temperature and a fever; it is considered that the set point is elevated, as determined by the posterior hypothalamus. At the onset of a fever, the body invokes heat-conservation mechanisms (such as shivering and cutaneous vasoconstriction) in order to raise the body temperature to its new, regulated stable temperature [Cooper et al. 1982]. In contrast, during exercise in heat, which may result in an increase in body temperature, body temperature rises to a new stable level where it is regulated by the hypothalamus, and only heat-dissipation mechanisms are invoked. Once a fever is induced, the elevated body temperature appears to be normally controlled by the usual physiologic processes around its new and higher regulated level [Taylor et al. 2008].
4.1.2 Muscular Activity and Work Capacity
The muscles are by far the largest single group of tissues in the body, representing some 45% of body weight. The bony skeleton, on which the muscles operate to generate their forces, represents a further 15% of body weight. The bony skeleton is relatively inert in terms of metabolic heat production. Even at rest, the muscles produce about 20% to 25% of the body’s total heat production [Rowell 1993]. The amount of metabolic heat produced at rest is quite similar for all individuals when it is expressed per unit of surface area or of lean or fat-free body weight. On the other hand, the heat produced by the muscles during exercise can be much higher and must be dissipated if a heat balance is to be maintained. The heat load from metabolism is therefore widely variable, and working in hot environments (which imposes its own heat load or restricts heat dissipation) poses the greatest challenge to normal thermoregulation [Parsons 2003].
The proportion of maximal aerobic capacity (·V.O2 max) needed to do a specific job is important for several reasons. First, the cardiovascular system must respond with an increased CO, which, at levels of work up to about 40% ·V.O2 max, is brought about by an increase in both SV and HR. When maximum SV is reached, additional increases in CO can be achieved solely by increased HR until maximal HR is reached [McArdle et al. 1996b; Taylor et al. 2008]. These changes in the cardiovascular response to exercise are responsible for providing sufficient blood flow to muscle to allow for the increase in muscular work [McArdle et al. 1996b]. Further complexities arise when high work intensities are sustained for long periods, particularly when work is carried out in hot surroundings [Åstrand et al. 2003]. Second, muscular activity is associated with an increase in muscle temperature, which then is associated with an increase in core temperature, with attendant influences on the thermoregulatory controls. Third, at high levels of exercise, even in a temperate environment, the oxygen supply to the tissues may be insufficient to completely meet the oxygen needs of the working muscles [Taylor et al. 2008].
In warmer conditions, an adequate supply of oxygen to the tissues may become a problem even at moderate work intensities because of competition for blood distribution between the working muscle and the skin [Rowell 1993]. Because of the lack of oxygen, the working muscles must begin to draw on their anaerobic reserves, deriving energy from the oxidation of glycogen in the muscles [McArdle et al. 1996b]. That leads to the accumulation of lactic acid, which may be associated with the development of muscular fatigue. As the proportion of ·V.O2 max used increases further, anaerobic metabolism assumes a relatively greater proportion of the total muscular metabolism. An oxygen “debt” occurs when oxygen is required to metabolize the lactic acid that accumulates in the muscles. This debt must be repaid during the rest period. In hot environments, the recovery period is prolonged as both the heat and the lactic acid stored in the body must be eliminated and water loss must be replenished. Generally, a euhydrated (normal hydration) state can be reached readily with normal ad libitum consumption of nonalcoholic beverages [Montain and Cheuvront 2008]. However, if a person consumes alcoholic beverages after a long day of hot work, the diuretic properties of alcohol may cause dehydration [Schuckit 2011]. This may delay the return to a euhydrated condition until the following day. There are also so-called alactate (absence of lactic acid accumulation) components to the oxygen debt and recovery process. Some of these alactate components involve blood returning to the lungs after distribution to the muscle, release of oxygen bound to myoglobin, residual effects of thermogenic hormones (epinephrine, norepinephrine, thyroxine, and glucocorticoids), and replenishment of adenosine triphosphate (ATP) and creatine phosphate (PCr) within the muscle cells [McArdle et al. 2010a].
It is well established that in a wide range of cool to warm environments, 5°C to 29°C (41°F–84.2°F), the deep body temperature rises during exercise to a similar equilibrium value in subjects exercising at the same proportion of ·V.O2 max [Lind 1976, 1977]. However, two individuals doing the same job and working at the same absolute load level who have widely different ·V.O2 max values will have quite different core temperatures. Current recommendations for an acceptable proportion of ·V.O2 max for daily industrial work vary from 30% to 40% of the ·V.O2 max, which, in comfortably cool surroundings [Åstrand et al. 2003], is associated with rectal temperatures of, respectively, 37.4°C to 37.7°C (99.3°F–99.9°F), whereas work at 50% ·V.O2 max yields a rectal temperature of 38°C (100.4°F) in the absence of heat stress.
In addition to sex- and age-related variability, the interindividual variability of ·V.O2 max is high; in a diverse worker population the range of ·V.O2 max that includes 95 of every 100 individuals is ±20% of the mean ·V.O2 max value. Differences in body weight (particularly the muscle mass) can account for about half that variability, but the source of the remaining variation has not been identified. Age is associated with a reduction in ·V.O2 max after its peak at about 20 years of age; in healthy individuals, the ·V.O2 max falls by nearly 10% each decade after age 30. The decrease with age is less in individuals who have maintained a higher degree of physical fitness. Women’s ·V.O2 max average about 70% of those for men in the same age group, because of lower absolute muscle mass, higher body fat content, and lower hemoglobin concentration [Åstrand and Rodahl 1977; Åstrand et al. 2003]. Many factors affect deep body temperature when men and women of varying body weights, ages, and work capacities do the same job.
Other sources of variability when individuals work in hot environments are differences in circulatory system capacity, sweat production, and ability to regulate electrolyte balance, each of which may be large.
Work capacity is reduced to a limited extent in hot surroundings if body temperature is elevated. That reduction becomes greater as body temperature increases. The ·V.O2 max is not reduced by dehydration itself (except for severe dehydration), so its reduction in hot environments seems to be principally a function of body temperature. Core temperature must be above 38°C (100.4°F) before a reduction is noticeable; however, a rectal temperature of about 39°C (102.2°F) may result in some reduction of ·V.O2 max.
The capacity for prolonged exercise of moderate intensity in hot environments is adversely affected by dehydration, which may be associated with a reduction of sweat production and a concomitant rise in rectal temperature and HR. If the total heat load and the sweat rate are high, it is increasingly more difficult to replace the water lost in the sweat (750–1,000 mL·h-1). The thirst mechanism is usually not strong enough to drive one to drink the large quantities of water needed to replace the water lost in the sweat [DOD 2003]. Evidence shows that as body temperature increases in a hot working environment, endurance decreases.
Cognitive function of individuals exposed to physical activity in a hot environment may increase, decrease, or change very little [O’Neal and Bishop 2010]. If cognitive function is impaired as the environmental heat stress increases, psychomotor, vigilance, and other experimental psychological tasks may show decrements in performance [Givoni and Rim 1962; Ramsey and Morrissey 1978; Hancock 1981, 1982; Marg 1983]. The decrement in performance may be at least partly related to increases in core temperature and dehydration. In some cases of heat exhaustion the rectal temperature is raised to the range of 38.5°C to 39.0°C (101.3°F–102.2°F), and disorganized central nervous system activity, as evidenced by poor motor function, confusion, increased irritability, blurring of vision, and changes in personality are present. These observations have prompted the unproven suggestion that reduced oxygen supply to the brain, called cerebral anoxia may be responsible [Macpherson 1960; Leithead and Lind 1964; Hancock 1982].
4.1.3 Circulatory Regulation
The autonomic nervous system and endocrine system control the allocation of blood flow among competing organ systems. The circulatory system delivers oxygen and nutrients to all tissues and for transports unwanted metabolites and heat from the tissues. However, as work in heat continues, the heart reaches a point where it cannot provide enough CO to meet both the peak needs of all of the body’s organ systems and the need for dissipation of body heat.
During exercise, widespread, sympathetic circulatory vasoconstriction occurs initially throughout the body, even in the cutaneous bed. The increase in blood supply to the active muscles is ensured by the action of locally produced vasodilator substances, which also inhibit (in the blood vessels supplying the active muscles) the increased sympathetic vasoconstrictor activity. In inactive vascular beds, there is a progressive vasoconstriction with the severity of the exercise. This is particularly important in the large vascular bed in the digestive organs, where venoconstriction also permits the return of blood sequestered in its large venous bed, allowing up to one liter of blood to be added to the circulating volume [Rowell 1977, 1993].
If the need to dissipate heat arises, the autonomic nervous system reduces the vasoconstrictor tone of the cutaneous vascular bed, followed by “active” dilation by an unknown mechanism. The sweating mechanism and an unknown critical factor that causes the importantly large dilation of the peripheral blood vessels in the skin are mutually responsible for humans’ large thermoregulatory capacity in the heat.
When individuals are exposed to continuous work at high proportions of ·V.O2 max or to continuous work at lower intensities in hot surroundings, the cardiac filling pressure remains relatively constant, but the central venous blood volume decreases as the cutaneous vessels dilate. The SV falls gradually and the HR must increase to maintain the CO. The effective circulatory volume also decreases, partly because of dehydration from sweating, and partly as the thermoregulatory system tries to maintain adequate circulation to the exercising muscles and the skin [Rowell 1977].
One of the most important roles of the cardiovascular system in thermoregulation is the circulation of warm blood from the body core to the skin for heat transfer to the environment. When the body is at rest, in the absence of heat strain, the skin blood flow is approximately 200 to 500 mL·min-1, but it can increase up to 7–8 L·min-1 when under high heat strain. The skin blood flow responds to changes in body core temperature and is required to mobilize warm blood from the body core to the periphery to transfer heat to the environment [Taylor et al. 2008]. However, the redistribution of blood flow to the skin results in a corresponding decrease in splanchnic, renal, and muscle blood flow. During exercise in the heat, blood is simultaneously required to supply oxygen to working muscle and to carry heat from the body core to the periphery (skin). However, muscle blood flow decreases by about 25% under heat strain, from approximately 2.4 mL·g-1·min-1 down to 2.1 mL·g-1·min-1, because of increased cutaneous demands [Taylor et al. 2008]. This is readily accomplished in a well-hydrated individual under compensable heat stress [González-Alonzo et al. 2008; Taylor et al. 2008]. In the dehydrated individual working in the heat, increased core body temperature imposes stress on the cardiovascular system, as well as the thermoregulatory system in the hypothalamus [Taylor et al. 2008]. The mechanism of redistribution of blood to muscle and to the cutaneous circulation, under conditions of dehydration secondary to sweating, leads to an effective contraction of plasma volume [González-Alonzo et al. 2008]. A decrease in an effective plasma volume can result in an increase in heart rate and myocardial oxygen demand [Parsons 2003]. During heat stress at both rest and exercise, the HR, CO, and SV all increase to a greater extent at a given workload than would normally be observed under thermoneutral conditions [Rowell 1993]. However, this cannot be sustained indefinitely or when the body is dehydrated from sweating or substantial blood flow is redistributed to the cutaneous circulation because they effectively reduce pressure volume and, therefore, SV and CO [Taylor et al. 2008].
4.1.4 The Sweating Mechanism
In a hot environment, where heat transfer by radiation is not possible, the primary means for the transfer of heat to the environment is evaporative heat loss through the vaporization of sweat from the skin. The sweat glands are found in abundance in the outer layers of the skin. They are stimulated by cholinergic sympathetic nerves and secrete a hypotonic watery solution onto the surface of the skin. Several other mechanisms for heat transfer to the environment include convection, conduction, and behavioral (e.g., leave the area, put on or take off clothes, drink water, or modify environmental controls) [Taylor et al. 2008]. In a hot environment that has an ambient wet-bulb temperature of 35°C (95°F) the body at rest can sweat at a rate that results in a body fluid loss of 0.8 to 1.0 L·h-1. For every liter of water that evaporates, 2,436 kJ (580 kcal) are extracted from the body and transferred to the environment [McArdle et al. 1996a]. The enormous capacity for heat loss through evaporation is generally more than adequate to dissipate metabolic heat generated by a subject at rest (~315 kJ·h-1for a 75-kg man) and at high levels of activity. The mean sweat rate in endurance athletes ranges from 1.5 to 2.0 L·h-1, which provides an evaporative heat loss capacity of 3,654 to 4,872 kJ that is about 11.6–15.5 times the amount of heat produced at rest [Gisolfi 2000]. This is generally more than adequate to remove heat from the body, even at extreme levels of metabolic heat production.
However, in environments with high humidity, even though sweating continues (increasing the level of dehydration), the evaporation of sweat is inhibited, heat transfer from the body is reduced, and the internal body temperature increases. Thus, when the heat index is greater than 35°C (95°F), largely due to high relative humidity (RH) (or with a WBGT of 33°C to 25°C [91.4°F to 77°F], depending on the workload), the evaporative heat loss is virtually nonexistent. Consequently, even if the ambient dry temperature is within a comfortable range (e.g., 23°C [73.4°F]), the high humidity could result in an “apparent temperature” or heat index high enough to cause heat stress for the worker and possible heat injury [Taylor et al. 2008].
Sweating results in significant dehydration, which leads to thermal and cardiovascular strain. People who are acclimatized to the heat lose water at a peak rate of 3 L·h-1 through sweating and may lose up to 12 L·h-1 during intense exercise in hot environments [McArdle et al. 1996a]. Thus, a major issue resulting from high heat stress is the need for adequate rehydration in order to replace the water lost to the environment from sweating and to reduce the risk of hyperthermia.
A rule of thumb is that a 0.45-kg (1.0-lb) decrease in body weight represents a 450-mL (15.2-oz) decrease in body water in extracellular and intracellular compartments that needs to be replaced by consumption of water. Another source of body water loss is the respiratory tract [McArdle et al. 1996a]. Average water loss from the respiratory tract at rest is about 350 mL per day under mild conditions of heat and humidity. Respiratory water loss will also contribute to the dehydration of the worker, and fluid loss increases with activity.
An important constituent of sweat is salt, or sodium chloride. In most circumstances in the United States, a salt deficit does not readily occur because the normal American diet provides 4 grams per day (174 mEq per day) of sodium [Food and Nutrition Board, Institute of Medicine 2004]. However, the sodium content of sweat in unacclimatized individuals may range from 10 to 70 mEq per day sweat (0.23–1.62 g·L-1) [Montain and Cheuvront 2008], whereas for the acclimatized individual, sodium lost to sweat may be reduced to 23 mEq·L-1 (0.530 g·L-1), less than 50% of that of the unacclimatized individual. It is possible for a heat-unacclimatized individual who consumes a restricted salt diet to develop a negative salt balance. In theory, a prolonged negative salt balance with a large fluid intake may result in a need for moderate supplementation of dietary salt. If there is a continuing negative salt balance, acclimatization to heat is diminished. However, salt supplementation of the normal diet is rarely required, except possibly for heat-unacclimatized individuals during the first 2 or 3 days of heat exposure [Lind 1976; DOD 2003]. By the end of the third day of heat exposure, a significant amount of heat acclimatization will have occurred, and salt loss in the sweat and urine and the need for salt in the diet are decreased. In view of the high incidence of elevated blood pressure in the U.S. worker population and the relatively high salt content of the average U.S. diet, even for those who watch salt intake, recommending an increase in salt intake is probably not warranted. Salt tablets can irritate the stomach and should not be used [DOD 1980; 2003]. Heavier use of salt at meals has been suggested for heat-unacclimatized workers during the first 2 or 3 days of heat exposure if they are not on a restricted salt diet by order of the responsible healthcare provider [DOD 2003]. Sodium can also be replenished by drinking fluids containing approximately 20 mEq·L-1 sodium, which is an amount found in many sports drinks [Montain and Cheuvront 2008]. In general, simply adding salt to the diet will adequately restore electrolyte balance. Moreover, carefully induced heat acclimatization reduces or eliminates the need for salt supplementation of the normal diet.
Because potassium is lost in sweat, it can be substantially depleted when unacclimatized workers suddenly have to work hard in hot climates; marked depletion of potassium can lead to serious physiologic consequences, including the development of heat stroke [Leithead and Lind 1964]. High table salt intake may increase potassium loss. However, potassium loss is usually not a problem, except for individuals taking diuretics, because potassium is present in most foods, particularly meats and fruits [Greenleaf and Harrison 1986]. Since some diuretics cause potassium loss, workers taking such medication while working in a hot environment should seek medical advice, telling their doctor that they work in the heat and asking how medications may affect how their body responds to the hot environment. Salt and potassium supplements, if recommended by the responsible healthcare provider, may only be needed during the acclimatization period or under extraordinary circumstances.
4.1.4.1 Water and Electrolyte Balance and the Influence of Endocrines
It is imperative to replace the water lost in the sweat. It is not uncommon for workers to lose 6 to 8 L of sweat during a working shift in hot industries. If the lost water is not replaced, then body water levels will progressively decrease, which shrinks the extracellular space, interstitial and plasma volumes, and water in the cells. Evidence supports that the amount of sweat production depends on the state of hydration [Leithead and Lind 1964; Henschel 1971; Greenleaf and Harrison 1986], so that progressive dehydration results in a lower sweat production and a corresponding increase in body temperature, which can be a dangerous situation.
Water lost in large quantities of sweat is often difficult to replace completely as the day’s work proceeds, and it is not uncommon for individuals to register a water deficit of 2% to 3% or greater of their body weight. During exercise in either cool or hot environments, a correlation has been reported between the elevation of rectal temperature and the percentage of water deficit in excess of 3% of body weight [Kerslake 1972 (p. 316)]. Because the normal thirst mechanism is not sensitive enough to ensure a sufficient water intake [Greenleaf and Harrison 1986; DOD 2003], every effort should be made to encourage individuals to drink water or other fluids (e.g., sports drinks). The fluid should be as palatable as possible, at less than 15°C (59°F). Small quantities taken at frequent intervals is a more effective regimen for practical fluid replacement than the intake of large amounts of fluids per hour [McArdle et al. 2010b]. Individual, not communal, drinking cups should be provided. Individuals are seldom aware of just how much sweat they produce or how much water is needed to replace that lost in the sweat; 1 L·h-1 is a common rate of water loss. With suitable instruction on how much to drink, most individuals will comply. A general rule of thumb for those exercising in the heat for 1 to 2 hours is to drink plain, cool water. Sweat is hypotonic to the plasma, and one does not lose a significant amount of sodium in the first hour or two of exercise [McArdle et al. 1996b]. Therefore, one does not require fluids containing electrolytes for this exposure. However, during prolonged sweating lasting several hours, it is advisable to consume a sports drink that contains balanced electrolytes to replace those lost during sweating, as long as the concentration of electrolytes/carbohydrates does not exceed 8% by volume. Exceeding the 8% limit will slow absorption of fluids from the gastrointestinal (GI) tract [Parsons 2003]. Since thirst is a poor indicator of hydration status, fluids should be consumed at regular intervals to replace water lost from sweating [McArdle et al. 1996b].
Plasma electrolytes (primarily sodium, potassium, and chlorine) are very strongly regulated by physiologic mechanisms because of their high importance in cellular volume and function. The control is accomplished by the kidney and is influenced by several hormonal pathways, including the renin-angiotensin-aldosterone system (RAAS) as well as antidiuretic hormone (ADH). Normal plasma sodium (Na+) concentration falls within the range of 135 to 145 mmol·L-1. Thus, hyponatremia is defined as a Na+ concentration <135 mmol·L-1 (mild) and severe hyponatremia as a plasma Na+ concentration of 120 mmol·L-1. Hyponatremia (low plasma sodium) is regarded as the consequence of a poor fluid-replacement strategy before, during, and after prolonged exercise and may become a potentially life-threatening condition. However, consumption of large quantities of water alone is not enough to cause hyponatremia. The condition is accompanied by significant loss of Na+ from sweating [Montain et al. 2006].
Hyponatremia usually occurs in high-endurance athletes participating in marathons, ultra-marathons, and so-called Iron Man triathlons, but it can also occur in people working for long periods of time in hot environments [Rosner and Kirven 2007]. The incidence of hyponatremia in high-endurance athletes ranges from 13% to 18%. Symptoms of hyponatremia can range from none to minimal (~70% of cases) to severe, including encephalopathy, respiratory distress, and death. During these events or work periods, hyponatremia develops when the athlete or worker consumes too much plain water in an attempt to rehydrate after copious sweating. The excess water results in a dilution of plasma Na+, which, in turn, causes an osmotic disequilibrium that can lead to cerebral edema (brain swelling) and pulmonary edema. These conditions can be fatal in a small number of patients [Rosner and Kirven 2007].
Risk factors for the development of hyponatremia are an exercise duration of >4 hours, gender (females are more likely to develop hyponatremia), low body mass, excessive consumption of water (>1.5 L·h-1), pre-exercise hydration, consumption of nonsteroidal anti-inflammatory medications (although not all studies have shown this), and extreme environmental temperature [Rosner and Kirven 2007]. Consumption of large quantities of water is highly correlated with the development of hyponatremia [Almond et al. 2005], the majority of athletes or workers in hot environments proceed to develop hyponatremia; however, not all those with hyponatremia become symptomatic [Rosner and Kirven 2007]. Therefore, the presence of mild hyponatremia may not be harmful. Although hyponatremia can have devastating consequences, such as death, for the sufferer and the pathophysiology of the condition involves multiple factors including the environmental as well as individual predisposition, the condition can be treated effectively to reduce the level of morbidity and mortality [Rosner and Kirven 2007]. Prevention of this condition involves appropriate use of fluid-replacement strategies.
Two hormones are important in thermoregulation: ADH and aldosterone. A variety of stimuli encourage the synthesis and release of those hormones, such as changes in plasma volume and plasma concentration of sodium chloride. ADH is released by the pituitary gland, which has direct neural connections with the hypothalamus but may receive neural input from other sources. Its function is to reduce water loss by the kidney, but it has no effect on the water loss through sweat glands. Body water, including the plasma volume in the vascular compartment, is also controlled by the RAAS. Changes in fluid volume or electrolyte (sodium) concentration will activate the RAAS to conserve fluids and electrolytes at the level of the kidney and sweat glands through the action of aldosterone [Jackson 2006]. The control of fluid (especially plasma) volume is also important in the maintenance of blood pressure and organ perfusion. Another product of the RAAS is angiotensin II, a powerful vasoconstrictor that helps maintain blood pressure and overall cardiovascular function in the presence of significant fluid loss from the vascular compartment, as well as a significant stimulator of the release of aldosterone from the adrenal glands [Williams et al. 2003].
4.1.4.2 Dietary Factors
A well-balanced diet in temperate environments usually suffices for hot climates. A very high protein diet might increase the urine output needed for nitrogen removal and increase water intake requirements [Greenleaf 1979; Greenleaf and Harrison 1986]. The importance of water and salt balance has been emphasized in the previous discussion, and the possibility that it might be desirable to supplement the diet with potassium has also been discussed. In some countries where the normal diet is low or deficient in vitamin C, supplements may enhance heat acclimatization and thermoregulatory function [Strydom et al. 1976]. Sodium supplements can be abused in the interest of increasing fluid retention. However, an excess of dietary sodium may actually decrease plasma volume, even with a controlled fluid intake. Therefore, supplemental dietary sodium must be used judiciously to prevent further dehydration and electrolyte depletion in workers [McArdle et al. 1996a; Williams et al. 2003]. In addition, certain dietary supplements may impair or alter cellular and systemic adaptions associated with both thermotolerance and heat acclimation in exercising humans [Kuennen et al. 2011].
4.1.4.3 Gastrointestinal Factors
Exertional hyperthermia may result in changes in GI permeability (“leaky gut”) that can result in the release of paracellular endotoxins [Zuhl et al. 2014]. The release of these endotoxins can trigger the release of leukocytes, which can result in the further release of proinflammatory cytokines [Leon 2007]. This inflammatory cascade results in further damage to the organ systems. Certain factors increase the susceptibility to increased GI permeability and endotoxin damage, including age; dehydration; loss of electrolytes from sweating; cardiovascular disease; use of certain medications (e.g., diuretics, anticholinergics, and NSAIDs); and alcoholism. In addition, certain foods containing quercetin (e.g., capers, red onions, etc.) can increase hyperthermia-induced GI permeability and aggravate the effects of hyperthermia [Kuennen et al. 2011; Zuhl et al. 2014].
4.1.5 Acclimatization to Heat
When workers are exposed to hot work environments, they readily show signs of distress and discomfort, such as increased core temperatures and heart rates, headache or nausea, and other symptoms of heat exhaustion [Leithead and Lind 1964; WHO 1969; Kerslake 1972(p. 316); Wyndham 1973; Knochel 1974; Hancock 1982; Spaul and Greenleaf 1984; DOD 2003]. On repeated exposure to a hot environment, there is a marked adaptation in which the principal physiologic benefit appears to result from an increased sweating efficiency evidenced by earlier onset of sweating, greater sweat production, and lower electrolyte concentration) and a concomitant stabilization of the circulation. As a result, after daily heat exposure for 7 to 14 days, most individuals perform the work with a much lower core temperature and HR and a higher sweat rate (i.e., a reduced thermoregulatory strain) and with none of the symptoms that were experienced initially [Moseley 1994; Armstrong and Stoppani 2002; DOD 2003; Navy Environmental Health Center 2007; Casa et al. 2009; ACGIH 2014]. During that period, the plasma volume rapidly expands, so that even though the blood is concentrated throughout the exposure to heat, the plasma volume at the end of the heat exposure in acclimatized individuals is often equal to or greater than before the first day of heat exposure.
Acclimatization to heat is an example of physiologic adaptation, which is well demonstrated in laboratory experiments and field experience [Lind and Bass 1963; WHO 1969]. However, acclimatization does not necessarily mean that individuals can work above the Prescriptive Zone (see Glossary) as effectively as below it [Lind 1977].
Full heat acclimatization occurs with relatively brief daily exposures to working in the heat. It does not require exposure to heat at work and rest for the entire 24 hours; in fact, such excessive exposures may be deleterious because it is difficult for individuals without heat acclimatization experience to replace all of the water lost in sweat. The minimum exposure time for achieving heat acclimatization is at least 2 hours per day, which may be broken into 1-hour exposures [DOD 2003]. Some daily period of relief from exposure to heat, in air-conditioned surroundings, is beneficial to the well-being of the individuals, if for no other reason than that they find it difficult to rest effectively in a hot environment [Kerslake 1972 (p. 316)]. The level of acclimatization depends on the initial level of individual physical fitness and the total heat stress experienced by the individual [DOD 2003]. Thus, a worker who does only light work indoors in a hot climate will not achieve the level of acclimatization needed to work outdoors with the additional heat load from the sun or to do harder physical work in the same hot environment indoors. Increased aerobic fitness confers at least partial acclimatization to the heat because of the increased metabolic heat production that occurs during exercise. Physically fit individuals have a reduced incidence of heat injury or illness during exposure to hot environments [Tipton et al. 2008].
Failure to replace the water lost in sweat will slow or even prevent the development of the physiologic adaptations described. It is important to understand that heat acclimatization increases the sweating rate; therefore, workers will have an increased water requirement [DOD 2003; Navy Environmental Health Center 2007]. In spite of the fact that acclimatization will be reasonably well maintained for a few days of no heat exposure, absence from work in the heat for a week or more results in a significant loss in beneficial adaptations. However, heat acclimatization can usually be regained in 2 to 3 days upon return to a hot job [Lind and Bass 1963; Wyndham 1973]. Heat acclimatization appears to be better maintained by individuals who are physically fit [Pandolf et al. 1977].
The total sweat production increases with acclimatization, and sweating begins at a lower core temperature [DOD 2003]. Cutaneous circulation and circulatory conductance decrease with acclimatization, reflecting the reduction in the proportion of CO that must be allocated for thermoregulation because of the more efficient sweating mechanism. Also during acclimatization, cardiovascular stability is improved, heart rate is lowered, stroke volume is increased, and myocardial compliance is improved [DOD 2003]. It is clear, however, that during exercise in heat, the production of aldosterone increases to conserve salt from both the kidney and the sweat glands, whereas an increase in ADH conserves the amount of water lost through the kidneys. The increase in levels of aldosterone results in a lower concentration of sodium in the sweat and thus serves to limit sodium and fluid loss from the plasma during exercise in the heat [Taylor et al. 2008].
It is clear from the foregoing descriptions that sudden seasonal shifts and sudden increases in environmental temperature may result in thermoregulatory difficulties for exposed workers. At such times, cases of heat disorder may occur, even for acclimatized workers.
Acclimatization to work in hot, humid environments provides adaptive benefits that also apply in desert environments, and vice versa; the qualifying factor appears to be the total heat load experienced by the individual [DOD 2003]. An acclimatization plan should be implemented at all workplaces where workers are exposed to heat. A recent study presented 20 cases of heat-related illness or death among workers [Arbury et al. 2014]. In most of these cases, employers had no program to prevent heat illness, or the program was deficient. Acclimatization was the program element most commonly missing and most clearly associated with worker death.
For a summary on acclimatization, see Table 4-1.
4.1.6 Other Related Factors
Many factors can increase a worker’s risk of heat-related illness. Some of the factors are environmental, such as direct sun exposure, high temperatures, and humidity. Indoor radiant heat sources, like ovens and furnaces, also can increase the amount of heat in the environment. An indoor work environment can become a heat hazard if air conditioning is unavailable or ventilation is insufficient [Chen et al. 2003]. Other factors may be related to characteristics of each individual worker or an individual’s current status of health at the time of exposure to heat stress in a hot environment. Heat-related illness factors are presented in Figure 4-1.
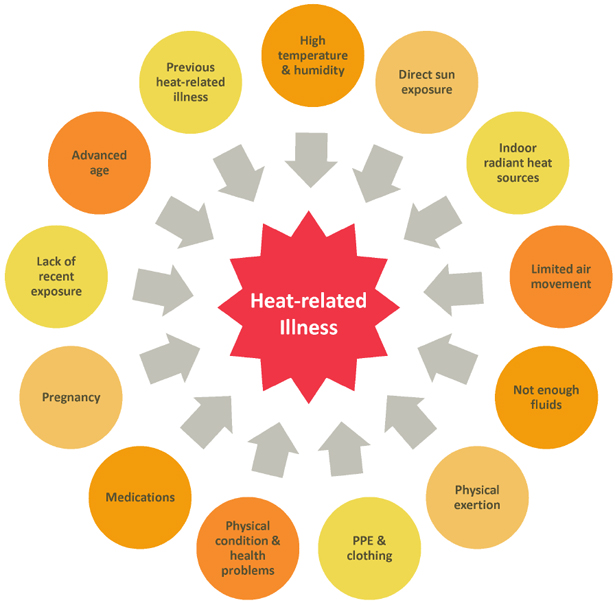
Figure 4-1. Examples of heat-related illness risk factors
4.1.6.1 Age
The aging process results in a more sluggish response of the sweat glands, which leads to a less effective control of body temperature in the sedentary individual [Taylor et al. 2008]. Aging also results in a decreased level of skin blood flow associated with exposure to heat. The cause remains undetermined, but the decrease in skin blood flow implies an impaired thermoregulatory mechanism, possibly related to reduced efficiency of the sympathetic nervous system [Hellon and Lind 1958; Lind 1977; Drinkwater and Horvath 1979]. For women, it has been found that the skin temperature increases with age in moderate and high heat loads but not in low heat loads [Hellon and Lind 1958; Drinkwater and Horvath 1979]. When two groups of male coal miners of average age 47 and 27 years, respectively, worked in several comfortable or cool environments, they showed little difference in their responses to heat near the REL with light work, but in hotter environments the older men showed a substantially greater thermoregulatory strain than their younger counterparts; the older men also had lower aerobic work capacities [Lind et al. 1970]. In analyzing the distribution of 5 years’ accumulation of data on heat stroke in South African gold mines, Strydom [1971] found a marked increase in heat stroke with increasing age of the workers. Thus, men over 40 years of age represented less than 10% of the mining population, but they accounted for 50% of the fatal cases and 25% of the nonfatal cases of heat stroke. The incidence of cases per 100,000 workers was 10 or more times greater for men over 40 years than for men under 25 years of age. In all the experimental and epidemiologic studies described above, the workers had been medically examined and were considered free of disease. Chronic hypohydration increases with age, which may be a factor in the observed higher incidence of fatal and nonfatal heat stroke in the older group. The reasons for hypohydration in older adults appear to be related to a decreased thirst drive resulting in, among other things, a suboptimal plasma volume. A reduced plasma volume (and most likely total body water, given the normal transfer of fluids between compartments) can impair thermoregulatory dynamics [McArdle et al. 2010a]. Another study suggests that age-related impairments in heat loss may not be evident during durations of <15 minutes of exercise; therefore, older workers may be safe from thermal strain and dehydration if they work for shorter intervals [Wright et al. 2014].
Older people are more susceptible to the effects of heat, and a significant fraction of those who suffer from heat disorders are older than 60 [Kenny et al. 2010]. The age-related susceptibility to heat is multifactorial and may be related to decreases in sweating and cutaneous blood flow, changes in cardiovascular function, and decreases in overall fitness [Kenney et al. 1990; Minson et al. 1998; Inoue et al. 1999]. Decreased sweating may be due to reduced sweat production rather than a reduced number of sweat glands [Inbar et al. 2004]. Thus, while acclimatization to heat can occur in the elderly, the rate of acclimatization is reduced [Armstrong and Kenney 1993; Inoue et al. 1999].
4.1.6.2 Sex
Although not all aspects of heat tolerance in women have been fully examined, their thermoregulatory capacities have been. An average-sized woman has a lower aerobic capacity than an average-sized man. When they work at similar proportions of their O2max, women perform either similarly or only slightly less well than men [Drinkwater et al. 1976; Avellini et al. 1980a; Avellini et al. 1980b; Frye and Kamon 1981].
A study examining sweat electrolyte loss during exercise in the heat found that sweat concentrations of Na+ and Cl- in men were higher than in women [Meyer et al. 1992]. The study was unable to explain why there appeared to be a difference between the sexes, although sweating rate and the effects of hormonal variations may play a part.
A recent study investigated whether there was an effect of sex on whole-body sudometer (a device to measure total sweat loss from the individual) activity during exercise in the heat [Gagnon and Kenny 2011]. Gagnon and Kenny found that females have a lower whole-body sweat response during exercise in the heat, which results in a greater increase in body temperature. The study concludes that the “results were not due to differences in physical characteristics, as both sexes were matched for body mass and surface area.”
According to Nunneley [1978], there seemed to be little change in thermoregulatory capacities at different times during women’s menstrual cycles.
Pregnancy is a consideration for women in coping with heat stress, and as it progresses tolerance of heat stress is reduced [Navy Environmental Health Center 2007]. Pregnancy naturally elevates the body’s temperature, which subjects women to heat exhaustion more rapidly during periods of extreme temperatures. The fetus acts as a source of metabolic heat and also increases the weight of the mother. Also, because the pregnant woman is caring for more than one body, more fluids and energy are needed to cool her core temperature. Increased heat loads during early pregnancy increase the fetus’ risk of teratogenic effects [Tillett 2011]. However, earlier studies suggested that the basal core temperature of pregnant women participating in submaximal exercise (i.e., bicycle test) studies over the course of their pregnancy decreased slightly postpartum [Lindqvist et al. 2003]. This result agrees with an even earlier study which reported that the magnitude of any first trimester exercise-associated thermal stress for the embryo or fetus is reduced by the maternal physiologic adaptions during pregnancy [Clapp 1991]. Moderate thermal stress (21.1°C [70°F] for 20 minutes) did not induce regular uterine contractility, nor was it harmful to the fetus when it was exposed during late pregnancy [Vaha-Eskeli and Erkkola 1991].
4.1.6.3 Body Fat
It is well established that obesity predisposes individuals to heat disorders [Leithead and Lind 1964]. In fact, heat disorders occur 3.5 times more frequently in the obese than in lean individuals [Henschel 1967; Chung and Pin 1996; Kenny et al. 2010]. The acquisition of fat means that additional weight must be carried, thereby calling for a greater expenditure of energy to perform a given task and use of a greater proportion of the ·V.O2 max on an overall per-weight basis. However, there is no difference in ·V.O2 max between obese and lean subjects if measured on an oxygen consumption per lean body weight basis [Vroman et al. 1983]. In addition, the ratio of body surface area to body weight (m2 to kg) in the obese individual becomes less favorable for heat dissipation. Probably more important is the lower physical fitness and decreased maximum work capacity and cardiovascular capacity frequently associated with obesity. The increased layer of subcutaneous fat provides an insulation barrier between the skin and the deep-lying tissues. The fat layer theoretically reduces the direct transfer of heat from the muscles to the skin [Wells and Buskirk 1971].
The limited number of studies in the area of heat stress and obesity has shown that obese individuals have a lower forearm blood flow during exercise in the heat, which is thought to reduce the cutaneous exchange of heat with the environment [Vroman et al. 1983; Kenny et al. 2010]. The reason for this occurrence is not completely clear, but it may be due to changes in sympathetic control over the vasculature or reduced stroke volume that regulate the relative blood flow to muscle (to perform work) and the blood flow to the cutaneous vasculature for the purpose of heat exchange. Some evidence exists that obese individuals suffer from asymptomatic small-fiber neuropathies, which lower thermal sensitivity [Herman et al. 2007].
It has also been proposed that the increase in thermal load in the obese is due simply to the reduced specific heat capacity of adipose tissue, which contains a lower amount of water per gram, in comparison with lean mass. Thus, for a given thermal load, the obese individual will store thermal energy at a greater rate than the lean individual, resulting in a greater average tissue temperature [Henschel 1967; Kenny et al. 2010].
Finally, the extra weight carried by the obese versus lean individual results in an increase in metabolic energy for any given task. The increase in metabolic energy produced in the form of muscular work results in an increase in body temperature that must be exchanged, in comparison with a lean individual performing the same task in the same environment [Bar-Or et al. 1969; Kenny et al. 2010]. Therefore, because it is apparent that obesity places the individual at a significantly higher risk of suffering a heat-related illness at any given workload or environmental temperature than the risk for a lean individual, additional accommodations may be necessary.
4.1.6.4 Drugs
(1) Therapeutic Drugs
Many drugs prescribed for therapeutic purposes can interfere with thermoregulation [Khagali and Hayes 1983]. Some of these drugs are anticholinergic in nature or involve inhibition of monoamine oxidative reactions, but almost any drug that affects central nervous system activity, cardiovascular reserve (e.g., beta blockers), or body hydration could potentially affect heat tolerance. Cardioselective beta blockers (e.g., atenolol, betaxolol, metoprolol, and acebutolol) do not allow the small blood vessels in the skin to dilate, thereby reducing blood flow, which impedes sweat production and causes body temperature to rise. Thus, beta blockers predispose those working in heat to heat-related emergencies. A worker who requires therapeutic medications should be under the supervision of the responsible healthcare provider who understands the potential ramifications of drugs on heat tolerance. In such instances, a worker taking therapeutic medications who is exposed only intermittently or occasionally to a hot environment should seek the guidance from the responsible healthcare provider. See Table 4-2 for additional information on proposed mechanisms of action of drugs implicated in intolerance of heat.
(2) Alcohol and Caffeine
It is hard to separate drugs used therapeutically from those used socially. Alcohol use (combined with heat stress) commonly has been associated with the occurrence of heat stroke [Leithead and Lind 1964]. It is a drug which interferes with central and peripheral nervous function and is associated with dehydration by suppressing ADH production. The ingestion of alcohol prior to or during work in the heat should not be permitted because it reduces heat tolerance and increases the risk of heat-related illnesses.
There are many drugs other than alcohol that are used on social occasions and have been implicated in cases of heat disorder, sometimes leading to death [Khagali and Hayes 1983]. Caffeine may be considered a socially accepted drug found in common beverages and foods (eg., coffee, tea, soft drinks, energy drinks, cocoa, chocolate) and in some over-the-counter analgesics that are consumed worldwide to enhance alertness, reduce fatigue, enhance athletic performance, augment the effects of mild analgesics and for simple enjoyment [Undem 2006; Taylor et al. 2008]. Coffee is one of the most widely consumed beverages in the world, and contains caffeine, which has a mild diuretic effect and should not be provided to workers to replace fluids lost to sweating. Moreover, coffee is generally consumed as a hot beverage and has the potential to exacerbate heat stress. In the past, caffeine was considered to contribute to heat stress by reducing fluid volume and result in cardiovascular strain during exposure to the heat [Serafin 1996].
Recent studies present evidence that caffeine may have less effect on heat tolerance than previously suspected [Roti et al. 2006; Armstrong et al. 2007a; Ely et al. 2011]. Armstrong et al. [2007a] propose that caffeine consumption does not result in water-electrolyte imbalances and does not reduce exercise-heat tolerance. They also suggest that “caffeinated fluids contribute to the daily human water requirement in a manner that is similar to pure water.” Ely et al. [2011] similarly found that a caffeine dose of 9 mg·kg-1 did not substantially alter heat balance during work in a hot environment. Caffeine appeared not to interfere with dry heat gains or evaporative heat losses, and total caffeine levels of 9 mg·kg-1 or less (approximately the amount found in one cup of coffee) could be considered safe in hot, dry environments. Roti et al. [2006] concluded that there was no evidence that dehydration or impaired thermoregulation resulted from chronic caffeine ingestion prior to or during exercise in the heat.
Although these studies present evidence that caffeine use may be harmless and acceptable for those exerting themselves in a hot environment, water is still the preferred hydrating beverage for before, during, and after work. However, virtually any nonalcoholic beverage is better than drinking nothing during heat exposure. Further research is needed on the effects of large doses of caffeine consumed at one time and the differences between modes of caffeine delivery (i.e., capsules, various beverages, and solid food) [Armstrong et al. 2007a]. Caffeine-containing fluids are now marketed to the public as energy drinks. These drinks contain higher than normal doses of caffeine (more than what is found in a cup of coffee or soft drink) and have been used extensively among competitive athletes prior to participation in athletic events [Burke 2008].
The lethal oral dose for caffeine in humans has been reported to range between 18 and 50 grams [HSDB 2011]. Other than the diuretic effects, this dose of caffeine is capable of inducing cardiac arrhythmias [Undem 2006], which could be potentiated by heat stress (i.e., as a result of already existing cardiovascular strain). It seems that there would also be a tendency to drink several energy drinks just to alleviate thirst as they are often available in smaller containers with less liquid, thus inadvertently overdosing on caffeine.
4.1.6.5 Non-heat Disorders
It has long been recognized that individuals suffering from degenerative diseases of the cardiovascular system and other diseases, such as diabetes or simple malnutrition, are at increased risk when they are exposed to heat and when stress is imposed on the cardiovascular system. The outcome is readily seen during sudden or prolonged heat waves in urban areas where there is a sudden increase in mortality, especially among older individuals, who supposedly have age-related reduced physiologic reserves [Leithead and Lind 1964; Henschel et al. 1969; Ellis 1972; Kilbourne et al. 1982]. In prolonged heat waves, the mortality is higher in the early phase of the heat wave [Henschel et al. 1969; Ellis 1972]. While acclimatization may play a part in the decrease in mortality during the latter part of a prolonged heat wave, the increased death rate in the early days of a heat wave may reflect an “accelerated mortality,” with the most vulnerable more likely to succumb at that time rather than more gradually as a result of degenerative diseases.
Any sicknesses or diseases in which GI permeability is compromised could result in greater susceptibility to leakage of endotoxin and the cascade of immune reactions and death that occur with heat stroke. For example, quercetin ingestion during heat acclimation prevented many of the benefits of heat acclimation, whereas glutamine ingestion improved GI barrier function and prevented endotoxin absorption and GI distress [Zuhl et al. 2014].
4.1.6.6 Individual Variation
In all experimental studies of the responses of humans to hot environmental conditions, a wide variation in responses has been observed. These variations are seen not only between individuals but also, to some extent, in the same individual exposed to high environmental stress on different occasions. Such variations are not totally understood. It has been shown [Wyndham 1973] that the influence of body size and its relationship to aerobic capacity in tolerance to heat could account for about half of the variability, leaving the remainder to be determined. Changes in hydration and salt balance might be responsible for some of the remaining variability [Buskirk and Bass 1980]. However, the degree of variability in tolerance to hot environments is poorly understood. Nevertheless, some individuals exhibit poor tolerance to heat and, of this group, some may have hormonal issues that disrupt their ability to control fluids and electrolytes which increases their susceptibility to heat injury [Rosner and Kirven 2007].
4.1.7 Heat-Related Illnesses and Work
The incidence of occupational heat-related illness in the United States is not well-documented by an existing surveillance system specific to occupational injury and illness. According to BLS data from 2010, 4,190 cases of illness and injury from exposure to environmental heat, resulting in one or more days of lost work, occurred among private industry and state and local workers [Bureau of Labor Statistics 2011]. In that same year, 40 workers died from exposure to environmental heat. Eighteen of these workers died in the construction industry; 6 workers died in the natural resources field (including agriculture) and mining; 6 died in professional and business services (including waste management and remediation); and 3 died in manufacturing. In addition, the National Fire Protection Association (NFPA) reported 2,890 cases of “thermal stress” in 2008, but this category includes both frostbite and heat exhaustion as per their definition [NIOSH 2010].
In the following sections, various worksites that may have heat exposure are evaluated and presented in short case study reports.
4.1.7.1 Health Hazard Evaluation (HHE) Reports
National Park
(The full report is available at http://www.cdc.gov/niosh/...)
Park management requested NIOSH to evaluate employees working in extreme heat (e.g., employees involved in maintenance of asphalt parking lots, housing repairs, grounds maintenance, or archaeological surveys), to review current and proposed heat stress management policies, and to recommend ways of preventing heat-related illnesses [NIOSH 2014b]. July temperatures in the park ranged from 31.1°C to 46.7°C (88°F to 116°F). Employees were asked to participate in work and medical history questionnaires, which also asked about health symptoms. Core body temperatures and heart rate were measured. Blood was analyzed daily for markers of muscle breakdown and dehydration during 4 workdays and the following 3 rest days. Estimates of how hard the workers were working were noted and daily temperatures and humidity were measured. The park’s heat stress policies and records of work-related injuries and illnesses were reviewed.
One worker was found to have a core body temperature above the ACGIH defined heat stress criteria (>38.5°C [101.3°F]); however, no workers were determined to be dehydrated or experiencing significant muscle breakdown. Several workers had sustained maximum heart rates. The heat stress policy was found to lack appropriate work/rest scheduling, and workers were not consistent in following the policy (i.e., failing to observe the buddy system rule).
The NIOSH HHE made the following recommendations for managers and workers:
Managers
- Avoid moderate to heavy outdoor tasks during summer months, or if necessary, work at night.
- Reduce the amount of time workers work in extremely hot weather.
- Revise the park’s heat stress policy to include work/rest schedules based on WBGT and workload.
- Require workers to conduct self- monitoring.
- Develop a workgroup (i.e., workers, responsible healthcare provider, and safety manger) to make decisions on self-monitoring options and standard operating procedures.
Workers
- Follow the heat stress policy.
- Carry a radio at all times.
- Avoid working alone (i.e., buddy system).
- Learn the signs and symptoms of heat-related illnesses.
- Self-monitor and document signs and symptoms of heat-related illnesses.
- Tell your supervisor if you have symptoms or if you note symptoms in a coworker.
- Drink plenty of fluids, and take rest breaks as needed.
- Volunteer to be on the work group to develop self-monitoring guidance for working in the heat.
Aluminum Smelter Potrooms
(The full report is available at http://www.cdc.gov/niosh/...)
NIOSH assessed workers’ exposure to heat while working in the potrooms at an aluminum smelter [NIOSH 2006a]. In the smelting process, alumina is reduced to nearly pure aluminum at an operating temperature of approximately 982.2°C (1,800°F). Workers were interviewed and completed questionnaires about their medical history, work history, and symptoms experienced during the shift on which they were monitored. Monitoring included core body temperature and heart rate. In addition, urine specific gravity and blood electrolytes were measured before and after shifts. WBGTs were monitored in several locations inside the potrooms, and outdoor weather conditions were monitored.
The mean outdoor temperature for the 5 days of evaluation was 25.6°C (78°F). The WBGT measurements ranged from 28.3° C to 48.9°C (83°F to 120°F), with dry bulb air temperatures reaching 56.7°C (134°F) and radiant temperatures reaching 86.7°C (188°F). High radiant heat means that the workers were absorbing rather than radiating heat, unless proper shielding was provided. Metabolic rates of employees were estimated to be light to moderate (115–360 watts). Except for the crane operator, portions of all tasks were found to exceed the NIOSH recommended ceiling limit (NOTE: the NIOSH recommended ceiling limit has been discontinued) and the ACGIH threshold limit value (TLV®) for working in a hot environment. Common symptoms reported during shifts included racing heartbeat or palpitations, headache, muscle cramps, and lightheadedness or dizziness. Post-shift values for blood bicarbonate, blood urea nitrogen (BUN), creatinine, and urine specific gravity increased significantly. Plasma volume depletion was suggested by the substantial decrease over the shift of the BUN to creatinine ratio and potassium level, and may have been caused by excessive sweating. Many of the workers were found to not be sufficiently hydrated. In addition, several participating workers had evidence of acute kidney injury, which may have been a result of or affected by volume depletion rhabdomyolysis caused by excess heat stress exposure or extreme physical activity.
The NIOSH HHE made the following recommendations for managers and workers:
Managers
- Reduce the physical demands on workers working in the potrooms.
- Require the use of heat-reflective personal protective equipment.
- Install cooling recovery areas in the potrooms.
- Do not use outdoor air to cool workers when it is over 35°C (95°F) outside.
- Follow the heat stress management program.
- Stop 8-hour overtime shifts during extremely hot weather.
Workers
- Use reflective personal protective equip- ment.
- Use the cooling recovery areas when on breaks.
- Take time to work safely.
Automobile Parts Manufacturing Facility
(The full report is available at http://www.cdc.gov/niosh/hhe/...)
The painting department of an automobile parts manufacturing facility was assessed in part for workers subjected to high heat [NIOSH 2003a]. WBGT monitors were placed in the loading and unloading area among the workers and in the cafeteria (for comparison) for the entire work shift. Heat stress was measured for six workers over 2 days, through the use of wireless devices that are swallowed and monitor core body temperature. In addition, heart rate and skin temperature were monitored with other devices. Pre- and post-shift body weights were measured on both days to determine degree of dehydration.
Four of the six participating workers exceeded the ACGIH core body temperature’s lower limit (38°C [100.4°F]) six times, and one worker exceeded its upper limit (38.5°C [101.3°F]) once. Of the 13 measurements taken over the 2 days in participating workers, nine showed signs of dehydration (post-weight was less than pre-weight). Three of these measures met or exceeded the 1.5% guideline for adequate hydration. The dry bulb temperatures ranged from 26.9°C to 30.1°C (80.5°F to 86.2°F) in the loading and unloading areas and 21.2°C to 21.5°C (70.2°F to 70.7°F) in the cafeteria. Inadequate ventilation was suspected by the steadily increasing temperature in the loading and unloading areas.
The NIOSH HHE made the following recommendations for managers:
- Allow workers to rest during the rest portion of the work/rest regiment, and do not assign any duties during this time.
- Position fans above workstations, not directly in front of the workers.
- Hire a consultant familiar with ventilation in hot processes to reduce heat.
Glass Bottle Manufacturer
(The full report is available at http://www.cdc.gov/niosh/hhe/...)
A manufacturer of glass containers for the beer, spirit, juice, and tea industries was assessed by NIOSH because of concern regarding heat-related illnesses among employees exposed to hot working environments in the hot end of the plant, including the forming department [NIOSH 2003b]. In this department, raw materials are melted together in a furnace at temperatures of 1260°C to 1537.8°C (2,300°F to 2,800°F). The manufacturer used various controls such as fans that supply cooler air from the basement, evaporative cooling fans, sports drinks, two 25-minute worker rest breaks (plus additional breaks at management’s discretion), and a review of heat safety during safety meetings and through displayed posters. WBGT measurements were collected in the forming department, metabolic rates of workers were estimated, and employees were interviewed.
The highest WBGT reading was 30.7°C (87.2°F), with a dry bulb temperature of 30.6°C (87.0°F) and a globe temperature of 46.5°C (115.7°F). These results indicated that most surfaces in the department were at an elevated temperature and acted as radiant heat sources. The nearby break room’s WBGT was 21.3°C (70.3°F), with a dry bulb temperature of 24.2°C (75.5°F). NIOSH guidelines were used to estimate the metabolic heat produced by the workers (186 kcal·h-1), resulting in a light workload rate. WBGTs and metabolic rates were then compared to those listed in the NIOSH RELs and ACGIH TLVs®, and both recommended a continuous work schedule in similar environments. Eighteen workers were interviewed, with two having experienced heat-related symptoms on a hot day a few months earlier (i.e. heart racing, lack of sweating, persistent headache). Other workers mentioned symptoms in previous years, including those related to heat exhaustion, cramping, and nausea. Some workers mentioned that new workers typically start work in June and are not given enough time to acclimatize, resulting in some quitting. In addition, workers noted that the fans were useful (particularly the evaporative coolers) but they were not well maintained and some were not functional.
The NIOSH HHE made the following recommendations for managers:
- Place the fans that supply cooler air from the basement and the evaporative cooling fans on a preventative maintenance schedule to ensure they are operational throughout the summer months.
- Develop a heat acclimatization program to decrease the risk of heat-related illnesses.
- Develop continuing education programs to ensure that all workers potentially exposed to hot environments and physically demanding job activities stay current on heat stress and heat stress prevention information.
- Monitor environmental heat exposures during the hottest months with a WBGT monitor at (or as close as possible to) the area where the workers are exposed.
- Establish criteria for the declaration of a heat alert.
- Develop a heat-related illness surveillance program, which includes establishing and maintaining accurate records of any heat-related disorder events and noting the environmental and work conditions at the time of disorder.
- Ensure that workers stay hydrated and do not lose more than 1.5% body weight during their shift.
- Create a buddy system so that workers can monitor each other for symptoms of heat disorders.
- Allow workers to take unscheduled breaks if they report feeling weak, nauseated, excessively fatigued, confused, and/or irritable during work in hot temperatures.
Truck Drivers
(The full report is available at http://www.cdc.gov/niosh/hhe/reports...)
An airline catering facility that included truck drivers was evaluated for ergonomic risk factors, heat and cold exposure, and job stress [NIOSH 2014a]. Although heat stress conditions in the food delivery trucks were not specifically evaluated, several employees and management representatives discussed temperatures in the trucks. Employees reported that the trucks were poorly maintained (e.g., some trucks had no air conditioning or had windows that did not open or close). Employees reported that the heating system in some of the trucks could not be turned off so it operated throughout the year.
Managers reported that a typical delivery job took about 2 hours and included loading the truck at the dock, driving approximately 25 minutes to the airport, going through airport security, driving to the plane parked on the tarmac, unloading carts from the truck to the plane, and then driving back to the dock. The delivery could take longer if the plane was not ready to receive the carts. Employees were expected to do three or four deliveries during their shift. Employees could take a break between deliveries in an air conditioned room at the airline catering facility. However, managers stated that on busy days employees sometimes skipped these breaks to keep up with job demands. Some employees also reported that they skipped breaks to shorten their shift so they could leave work early.
Drivers, loaders, and sanitation (i.e., autoclave operators, dishwashers) employees were potentially exposed to hot temperatures. Heat measurements in the autoclave area suggested a continuous work schedule is acceptable for acclimatized employees with moderate workloads when outdoor temperatures are in the mid-70s. For the temperatures measured in these work areas, acclimatization for most people occurred in 4 days by exposing them to progressively longer periods in a hot work environment. The company had heat-related training materials available. The training included sources of heat, symptoms of heat-related disorders, and recommendations for preventing heat stress, such as taking breaks in a cool area and drinking one glass of water every 20 minutes. However, breaks were limited to two per 8-hour shift, and beverages were prohibited in most work areas.
The NIOSH HHE made the following heat stress-related recommendations for managers and workers:
Managers
- Train workers on the health effects of exposure to hot temperatures and ways to be more comfortable at work. Inform employees and supervisors of OSHA heat safety tools found at https://www.osha.gov/heat/index.html. The website provides information on protective measures they can take on the basis of the heat index at the worksite.
- Develop and implement a heat stress prevention program. Establish mandatory breaks and access to fluids for workers exposed to heat.
- Ensure new trucks have air conditioning and repair systems in existing vehicles.
Workers
- Drink plenty of fluids when exposed to heat.
- Take regular breaks to recover from extreme temperatures.
- Take part in safety committees.
- Report symptoms to supervisors and medical staff as soon as they occur.
4.1.7.2 Case Studies
Landscaping Case Study
A 30-year-old male landscape mowing assistant collapsed and died of heat stroke after a day of caring for residential lawns [NIOSH 2002]. Two hours before his death he had complained of feeling light-headed and short of breath, but he refused assistance offered to him by his partner. The worker was on medication that had a warning about exposure to extreme heat, and this might have interfered with body temperature regulation. The landscape worker had been wearing two pairs of work pants on the day he died, but his partner did not notice any profuse sweating or flushed or extremely dry skin. Upon collapse, the victim was treated by emergency medical services (EMS) personnel at the site and then transported to the hospital. There he was pronounced dead, with an internal temperature of 42°C (107.6°F). On the day of the incident, the maximum air temperature was 17.2°C (81°F).
The following recommendations were made after the incident:
- Employers should ensure that supervisors/managers monitor workers during periods of high heat stress.
- Identify workers with risk factors that would predispose them to heat-related illnesses.
- Train workers about heat stress, heat strain, and heat-related illnesses.
- Ensure all workers are able to recognize the signs and symptoms of heat-related illnesses in themselves and in others.
- Stress the importance of drinking nonalcoholic beverages before, during, and after working in hot conditions.
- Periodically remind workers of the signs of heat-related illness and encourage them to drink copious amounts of water during hot conditions.
Migrant Farm Worker Case Studies
A male Hispanic worker aged 56 years died of heat stroke after hand-harvesting ripe tobacco leaves for 3 days on a North Carolina farm [CDC 2008]. On the third day, the man started working at 6:00 a.m. and took a short mid-morning break and a 90-minute lunch break. Mid-afternoon, a supervisor observed the man working slowly and reportedly instructed him to rest, but the man continued working. An hour later, the man appeared confused and coworkers carried him to the shade and tried to get him to drink water. The man was taken by ambulance to an emergency department, where his core temperature was recorded as 42.2°C (108°F) and, despite treatment, he died. On the day of the incident, the local temperature was approximately 33.9°C (93°F) with 44% RH and clear skies. The heat index (a measurement of how hot it feels when both actual temperature and RH are considered) was in the range of 30°C to 44.4°C (86°F to 112°F) that day.
In an additional, similar case study, a male Hispanic migrant worker aged 44 years died of heat stroke while working at another North Carolina tobacco farm [NIOSH 2006b]. He had been working in the fields for about the last week of July. On August 1, the heat index was between 37.8°C and 43.3°C (100°F and 110°F). Around 3 p.m. the worker complained to the crew leader that he was not feeling well. He drank some water and was driven to the workers’ housing and left alone. He was found unconscious approximately 45 minutes later. Emergency medical personnel responded within 5 minutes and the worker was taken to the hospital and pronounced dead. His core body temperature was recorded at 42.2°C (108°F).
The following recommendations were made after the incident:
- Agricultural employers should develop, implement, and enforce a comprehensive safety and health program that includes standard operating procedures for prevention of heat-related illnesses.
- Train supervisors and workers on how to prevent, recognize, and treat heat illness, using a language and literacy level that workers can understand.
- Establish a hydration program that provides adequate potable water (or other appropriate hydrating fluid) for each worker and which encourages workers to drink at regular intervals.
- Monitor environmental conditions and develop work/rest schedules to accommodate high heat and humidity.
- Provide an appropriate acclimatization program for new workers to a hot environment, workers who have not been on the job for a period of time, and experienced workers during a rapid change in excessively hot weather.
- Provide prompt medical attention to workers who show signs of heat-related illness.
Construction Case Study
A 41-year-old male construction laborer was sawing boards to make concrete forms that were to be part of an addition to a factory [NIOSH 2004]. At 5 p.m. the worker collapsed in the parking lot on the way to his vehicle. He was found 30 minutes later by a factory worker, who then returned to the factory and reported the situation to a supervisor. The receptionist was instructed to call EMS while the supervisor administered emergency care to the collapsed worker. The worker’s body temperature was recorded as 41.7°C (107°F) by the EMS and as 42.2°C (108°F) when admitted to the hospital. The worker died the next day from heat stroke.
The following recommendations were made after the incident:
- Train supervisors and workers to recognize symptoms of heat exhaustion/stroke when working in high heat index and/or humid conditions.
- To avoid dehydration and heat exhaustion/stroke, workers should be given frequent breaks and be provided drinking water and other hydrating drinks when working in humid or hot conditions.
- Work hours should be adjusted to accommodate environmental work conditions such as a high heat index and/or high humidity.
Fire Fighter Case Study
During the construction of a fire line during a small wildland fire, a 21-year-old fire fighter died from heat stroke, another was overcome by heat stroke and survived, and two others suffered heat exhaustion [NIOSH 1997]. The crew had initially started their day by exercising 1 to 1.5 hours as part of their physical training regimen. The 21-year-old had been sick with a suspected viral or bacterial infection in recent days. After physical training, the crew practiced constructing a fire line for about 1 hour. At 11:45 a.m., the crew arrived at the actual fire that had been reported and constructed a fire line as a precaution in an area with no fire. Crews carried canteens and could drink when desired. Around 1:45 p.m., the crew took a 15-minute break and drank water or Gatorade. At 2 p.m. they resumed the fire line, and 15 minutes later one member fell from heat exhaustion and broke his shoulder. He was administered first aid and transported to the hospital. Prior to this, a member of another crew suffered heat exhaustion, was treated by paramedics, and was returned to his base of operation. Around 2:30 p.m., the 21-year-old moved off the line as though he was going to relieve himself. However, 5 minutes later he was found on the ground thrashing. The crew leader found that he was semiconscious and suffering from heat stroke. His clothing was removed, water was dumped on his skin, and chemical cold packs were applied to his body. At 2:50 p.m. paramedics arrived and continued to administer aid. At 3 p.m. another crew member experienced symptoms of heat stroke. An ambulance transported the 21-year-old to the hospital at 3:20 p.m., but he died early the following morning. The maximum measured temperature during the incident was 36.7°C (98°F).
The following recommendations were made after the incident:
- Require supervisors to regularly medically monitor fire fighters, using generally accepted techniques, during periods of high heat stress.
- Ensure fire fighters’ workloads are appropriate for their level of acclimatization.
- Ensure fire fighters’ workloads are appropriate for the ambient weather conditions and clothing.
4.2 Acute Heat Disorders
Although heat disorders are interrelated and seldom occur as discrete entities, each has unique clinical characteristics [Minard and Copman 1963 (p. 253); Leithead and Lind 1964; Minard 1973; Lind 1977; Dinman and Horvath 1984; Springer 1985]. These disorders range from simple postural heat syncope (fainting) to the complexities of heat stroke. A common feature in all the heat-related disorders (except simple postural heat syncope) is some degree of elevated body temperature, which may be complicated by deficits of body water. The prognosis depends on the absolute level of the elevated body temperature, the promptness of treatment to lower the body temperature, and the extent of deficiency or imbalance of fluids or electrolytes. A summary of classification, clinical features, prevention, and first-aid treatment of heat-related illnesses is presented in Table 4-3.
4.2.1 Heat Stroke
Heat stroke can occur as either classic or exertional. Classic heat stroke includes (1) a major disruption of central nervous system function (unconsciousness or convulsions); (2) a lack of sweating; and (3) a rectal temperature in excess of 41°C (105.8°F) [Minard and Copman 1963 (p. 253); Leithead and Lind 1964; Shibolet et al. 1976; Khagali and Hayes 1983]. The 41°C (105.8°F) rectal temperature is an arbitrary value for hyperpyrexia because observations are made only after the admission of patients to hospitals, which may occur from about 30 minutes to several hours after the event. Exertional heat stroke occurs in physically active individuals who will often continue sweating [DOD 2003; Armstrong et al. 2007b; Navy Environmental Health Center 2007]. With exertional heat stroke, the skeletal muscle often rapidly breaks down, which is called acute rhabdomyolysis (see 4.2.1.1), and also results in renal failure [DOD 2003]. The risk of renal failure is about 25% for those suffering from exertional heat stroke [Navy Environmental Health Center 2007]. For additional comparisons between classic and exertional heat stroke see Table 4-4. The metabolic and environmental heat loads that give rise to heat stroke are highly variable and are often difficult or impossible to accurately reconstruct. Also, the medical outcomes vary among patients, depending on the caregiver’s knowledge, understanding, skill, and available facilities.
Heat stroke is a medical emergency, and rapidly cooling the affected worker is imperative. If possible, cool the worker quickly with an ice water bath. Placing the affected worker in a shady area, removing outer clothing and wetting or applying ice to the head, neck, armpits, and groin areas, and increasing air movement to enhance evaporative cooling are all important activities to perform while waiting for or transporting to medical care. A worker experiencing heat exhaustion or heat stroke should not be sent home or be left unattended without a specific order from a responsible healthcare provider.
Frequently, by the time a worker is admitted to a hospital, the disorder has progressed to a multisystem emergency affecting virtually all tissues and organs [Dukes-Dobos 1981]. In the typical clinical presentation, the central nervous system is disorganized and there is commonly evidence of fragility of small blood vessels, possibly coupled with the loss of integrity of cellular membranes in many tissues. The blood-clotting mechanism is often severely disturbed, as are liver and kidney functions. It is not clear, however, whether these events are present at the onset of the disorder or whether they develop over time. Postmortem evaluation indicates that few tissues escape pathological involvement. Early recognition of the disorder or its impending onset, when combined with appropriate treatment, considerably reduces the death rate and the extent of organ and tissue involvement [DOD 2003; Navy Environmental Health Center 2007].
4.2.1.1 Rhabdomyolysis
Rhabdomyolysis is a medical condition associated with heat stress and prolonged physical exertion, resulting in the rapid breakdown of muscle and the rupture and necrosis of the affected muscles. When muscle tissue dies, electrolytes and large proteins that formed the muscle’s contractile mechanism are released into the bloodstream [Khan 2009; Cervellin et al. 2010]. Potassium is the main electrolyte released into the blood by the death of muscle tissue, and high levels can cause irregular and dangerous heart rhythms and seizures. In addition, the large muscle proteins can damage the delicate filtration system of the kidneys.
Classic symptoms of rhabdomyolysis are muscle pain, cramping, swelling, weakness, and decreased range of motion of joints. One of the signs of rhabdomyolysis is dark or tea-colored urine [Brudvig and Fitzgerald 2007; Khan 2009; Cervellin et al. 2010]. However, symptoms can vary between individuals, with some experiencing nonspecific symptoms such as fatigue, exercise intolerance, abdominal pain, back pain, nausea or vomiting, and confusion, while others might have no symptoms [Huerta-Alardin et al. 2005; Brudvig and Fitzgerald 2007]. In one study, only half of patients with confirmed rhabdomyolysis reported muscle pain or weakness [Cervellin et al. 2010]. Because muscle cramps and dark urine after prolonged exertion may be the only symptom and sign, rhabdomyolysis may be mistaken for another heat-related illness and dehydration. This was confirmed by a study of cases in which rhabdomyolysis was initially misdiagnosed; it found that the most common diagnoses given were heat stress and dehydration [Gardner and Kark 1994]. Delays in recognizing rhabdomyolysis due to misdiagnosis are problematic. The more serious the case is and the longer the delay in making the correct diagnosis and initiating treatment, the greater the risk for increased complications, some of which may be permanent such as reduced or loss of kidney function due to compartment syndrome.
Rhabdomyolysis is diagnosed by measurement of creatine kinase (CK), also known as creatine phosphokinase (CPK), in the blood by a licensed health care professional. The severity of rhabdomyolysis depends upon damage to other organ systems and the peak CK level. Long-term health consequences of rhabdomyolysis vary widely and largely depend on speed of recognition and treatment [Line and Rust 1995]. Mild rhabdomyolysis can be treated by drinking lots of fluids [George et al. 2010]. Severe cases require hospitalization and aggressive treatment with intravenous fluids to dilute the proteins and thus minimize their damage to the kidney; monitoring of the heart for dangerous rhythm changes from the surge of electrolytes; and monitoring of kidney function [Sauret et al. 2002]. In severe cases, the kidneys may fail and immediate dialysis is needed to mechanically remove proteins and electrolytes from the blood [Bosch et al. 2009]. The drop in kidney function may be temporary, but in some cases kidney function does not recover, leaving a formerly healthy individual facing a lifetime of dialysis or possibly a kidney transplant. Up to 8% of cases of rhabdomyolysis are fatal [Cervellin et al. 2010]. In the past, rhabdomyolysis screening involved a urine test that was positive if it contained myoglobin but no red blood cells. However, because myoglobin is quickly excreted and levels may return to normal within 6 hours of muscle injury, it is not considered a reliable test for the condition. One study showed that only 19% of patients diagnosed with rhabdomyolysis had elevated urine myoglobin levels at the time of diagnosis [Counselman and Lo 2011].
Risk factors for rhabdomyolysis include elevated core body temperature from environmental heat, from heat generated by physical exertion, or from medical conditions that raise the body’s temperature (e.g., malignant hyperthermia); dehydration; prescription medications (e.g., cholesterol-lowering statins and antidepressants); over-the-counter medications (e.g., antihistamines, non-steroidal anti-inflammatory medications, omeprazole); excessive caffeine intake; use of dietary supplements (e.g., creatine and Hydroxycut™); use of medications, alcohol, or amphetamines; underlying medical conditions (e.g., sickle cell trait or lupus); and concurrent bacterial or viral infections (e.g., influenza, Epstein-Barr, or Legionella) [Wrenn and Oschner 1989; Line and Rust 1995; Huerta-Alardin et al. 2005; Melli et al. 2005; Do et al. 2007; Makaryus et al. 2007; Dehoney and Wellein 2009; Nauss et al. 2009; Chatzizisis et al. 2010; George et al. 2010; de Carvalho et al. 2011].
Differentiation between the types of heat exposure that led to the rhabdomyolysis is important because they have different signs, symptoms, and potential sequelae. Victims of classic heat stroke usually do not experience rhabdomyolysis as severe as those with exertional heat stroke. Individuals with exertional heat stroke with rhabdomyolysis often have higher levels of CK, presence of lactic acidosis, significant drop in blood calcium levels (while showing a dangerously high spike in blood potassium levels that can cause heart rhythm irregularities), increased incidence of kidney failure (20%–30% vs <5%), and increased risk for a potentially fatal coagulation disorder called disseminated intravascular coagulation [DOD 2003].
Some jobs intrinsically have risk factors for heat related illness and rhabdomyolysis, such as fire fighting. Fire fighters are at increased risk because they routinely encounter several heat sources as they do their jobs: heat from the fire, ambient heat when working during hot weather, and heat generated by prolonged physical exertion during fire-suppression efforts. Physical exertion is often increased by the effort of carrying additional weight. Structural fire fighters may carry an additional 40 to 60 pounds of turnout gear, self-contained breathing apparatus (SCBA) air tanks, and various equipment (e.g., ventilation hooks can weigh 20–80 lb). Although wildland fire fighters do not wear turnout gear because of the remoteness of the fires they fight, they must be able to be self-sufficient once they are at the fire location, which can mean carrying 40- to 110-lb packs while hiking over steep terrain for prolonged periods. In a 2011 investigation into the heat stroke death of a wildland fire fighter belonging to an interagency “hotshot crew.” NIOSH reviewed agency records and found that they had documented 255 nonfatal cases of heat-related illness in the previous 12 years [NIOSH 2012]. NIOSH investigated the exertional heat stroke death of a 26-year-old structural fire fighter who collapsed at the end of a 4.4-mile jog during his cadet training course in 22.8°C (73°F) ambient temperature. On arrival at the emergency department he had a core temperature of 40.7°C (105.3°F) and was found to have rhabdomyolysis. He subsequently developed acute kidney failure and disseminated intravascular coagulation before he died 5 days later.
The incidence of symptomatic exertional rhabdomyolysis in the general population is unknown [Alpers and Jones 2010]. Individuals in occupations that require prolonged, intense physical activity, such as military personnel/recruits, law enforcement, and athletes, are at increased risk for developing rhabdomyolysis [CDC 1990; Gardner and Kark 1994; Walsh and Page 2006; O’Connor and Duester 2011]. Most cases of acute exertional rhabdomyolysis among military trainees occur during the first week of training [Olerud et al. 1976]. More recent data, from the Armed Forces Health Surveillance Center, reported 378 cases of “rhabdomyolysis likely due to physical exertion or heat stress” in 2013 alone, representing a 33% increase in the annual incidence rate between 2009 and 2013. It was noted that 71% of these cases occurred between May and September [Armed Forces Health Surveillance Center 2014]. It is a recurrent issue during summer football practice, as this involves intense physical training regimens often in full protective gear during hot weather.
Repetitive exhaustive exercise regimens including “incentive training” with high-intensity repetitive exercises such as push-ups are more likely to lead to rhabdomyolysis than prolonged submaximal exercise regimens [Olerud et al. 1976]. Although it is not uncommon for individuals in the general population who engage in exertional activities higher than their baseline level of fitness to develop exertional rhabdomyolysis, it also occurs in highly conditioned individuals who may engage in supramaximal (i.e., high intensity, usually short duration) exercise or who have other risk factors concurrent with an exertional activity [Walsh and Page 2006].
Aside from addressing the cause of rhabdomyolysis (i.e., cool the body down if temperature is high), the common theme of treatment of rhabdomyolysis is fluids. Mild cases of rhabdomyolysis can be treated with oral rehydration fluids, which serve to flush the harmful proteins out of the blood before they can harm the kidneys while restoring the volume depletion that often accompanies rhabdomyolysis. Treatment of more severe cases of rhabdomyolysis require fluid intake rates for durations that cannot be accomplished by drinking, which is why inpatient treatment with intravenous fluids is necessary. The treatment goal is to obtain urine output of 200 to 300 mL per hour while monitoring serum CK, potassium, and kidney function markers. Patients with severe rhabdomyolysis should be placed on cardiac monitors, because the elevated levels of potassium can produce potentially fatal heart-rhythm abnormalities. Low blood calcium levels are common with exertional rhabdomyolysis and can result in seizures. Consultation with a nephrologist may be needed for emergency hemodialysis if the kidney function declines. Kidney function may or may not recover, and if not, the patient will require regular dialysis for the rest of her or his life.
Another potential serious complication of rhabdomyolysis is compartment syndrome, which can occur if the damaged muscle is inside a fibrous sheath with other muscles. Most of these sheaths are found in the arms and legs [Cervellin et al. 2010]. All muscles within the same sheath are considered to be in the same compartment. During rhabdomyolysis, the injured muscle becomes inflamed and swollen. Because the fibrous sheath of the compartment cannot expand, the pressure from this swelling can become severe enough to prevent blood from entering the sheath, endangering all muscles inside that compartment. Unless the sheath is cut open by a surgeon to relieve the pressure and restore blood flow, all the muscles in that compartment could die, resulting in permanent loss of function of the affected limb(s) [Walsh and Page 2006].
Patients with compartment syndrome may present with some of the classic “5 P’s” of vascular insufficiency: pain, pallor, pulselessness, paresthesias (i.e., sensation of tingling, numbness, or burning, usually felt in the hands, feet, arms, or legs), and paralysis. Pain is the most common of these symptoms and is often severe to the point of being difficult to control even with narcotic analgesics. This pain is worsened with passive extension, and onset may be delayed for hours to days after the initial injury because it can take time for the swelling of the damaged muscle to become severe enough to block the blood flow into the compartment. On examination, the affected limb is swollen, tense, and very tender to applied pressure (although compartment syndrome may occur in the abdomen and neck, it most often occurs in the lower extremities). Swelling of the affected area may also be evaluated by measurement of that extremity’s circumference and comparison with that of the unaffected side. However, if compartment syndrome is suspected, then immediate surgical consultation is indicated, along with measurement of the pressure within the compartment. Compartment pressures should not exceed 30 mmHg (4 kPa); when they do, it is an indication for immediate transfer to surgery for fasciotomy. Prompt treatment is critical because permanent damage to the muscle occurs when its blood flow is blocked for more than 8 hours [Haller 2011].
4.2.2 Heat Exhaustion
Heat exhaustion is often considered a precursor to the more serious heat stroke. This disorder has been encountered frequently in experimental assessment of heat tolerance. Usually, it is accompanied by a slightly elevated core body temperature (38°C–39°C, or 100.4°F–102.2°F). The symptoms of headache, nausea, vertigo, weakness, thirst, heavy sweating, irritability, and a decreased urine output are common to both heat exhaustion and the early stage of heat stroke. There is wide variation in the ability to tolerate an increased mean body temperature; some individuals cannot tolerate rectal temperatures of 38°C–39°C (100.4°F‒102.2°F) and others continue to perform well at even higher rectal temperatures [Joy and Goldman 1968].
Failure to replace water predisposes the individual to one or more of the heat disorders, especially heat exhaustion and heat stroke. Data suggest that cases of heat exhaustion can be expected to occur some 10 times more frequently than cases of heat stroke [Khagali and Hayes 1983].
4.2.3 Heat Cramps
Heat cramps are not uncommon in individuals who work hard in the heat. The exact cause or causes have not been determined, but heat cramps may be attributed to or associated with a continued loss of salt in the sweat, accompanied by a copious intake of water without appropriate replacement of salt. Other electrolytes, such as magnesium, calcium, and potassium, may also be involved. Cramps often occur in the muscles principally used during work and can be readily alleviated by rest, the ingestion of water, and the correction of any body fluid electrolyte imbalance (e.g., with sports drinks containing carbohydrates and electrolytes [serum sodium concentration <136 mEq·L-1]). Salt tablets should not be taken. Salt losses are best replaced by the ingestion of normal salted foods or fluids over many hours [DOD 2003].
4.2.4 Heat Syncope
Heat syncope (fainting) usually occurs with prolonged standing or sudden rising from a sitting or supine position; in such instances, temporary circulatory failure, due to pooling of blood in the peripheral veins, results in a decrease in diastolic filling of the heart [DOD 2003]. Symptoms of heat syncope include light-headedness, dizziness, and fainting. Factors that may contribute to heat syncope include dehydration and lack of acclimatization. Workers who have fainted will usually recover rapidly if they sit or lay down; however, complete recovery of stable blood pressure and HR may take an hour or two [DOD 2003].
4.2.5 Heat Rashes
The most common heat rash is prickly heat (miliaria rubra), which appears as red papules, usually in areas where clothing is restrictive, and gives rise to a prickling sensation, particularly as sweating increases. It occurs in skin that is persistently wetted by unevaporated sweat, apparently because the keratinous layers of the skin absorb water, swell, and mechanically obstruct the sweat ducts [Pandolf et al. 1980b, 1980a; DiBenedetto and Worobec 1985]. If untreated, the papules may become infected and develop secondary staphylococcal infections [DOD 2003]. Another skin disorder (miliaria crystallina) appears with the onset of sweating in skin previously injured at the surface, commonly in sunburned areas. The damage prevents the escape of sweat and results in the formation of small to large watery vesicles, which rapidly subside once sweating stops; the problem ceases to exist once the damaged skin is sloughed.
Miliaria profunda occurs when sweat ducts are blocked below the skin surface. This rash also occurs following a sunburn injury but has been reported to occur without clear evidence of previous skin injury. Discrete, pale elevations of the skin, resembling gooseflesh, are present.
In most cases, these rashes disappear with return to a cool environment. When a substantial part of the day is spent in cool and/or dry areas so that the skin surface can dry, the rashes are less likely to occur or occur with diminished frequency.
Although heat rashes are not dangerous in themselves, each can impair areas of skin and reduce sweating that reduces evaporative heat loss and impacts thermoregulation. Wet and/or damaged skin can also absorb toxic chemicals more readily than dry, unbroken skin. In experimentally induced miliaria rubra, sweating capacity recovered within 3 to 4 weeks [Pandolf et al. 1980b, 1980a].
4.3 Chronic Heat Disorders
Some long-term effects from heat stress (noted from anecdotal, historical, epidemiologic, and experimental evidence) have been suggested. Severe heat-related illness may cause permanent damage to a person’s organs, such as the heart, kidneys, and liver, which may result in a chronic disorder.
One study compared a cohort of U.S. Army personnel hospitalized for heat-related illness with those that had appendicitis. Those with heat-related illness had a 40% higher risk of all-cause mortality than the appendicitis patients [Wallace et al. 2007]. Furthermore, it was found that males with heat-related illness were at an increased rate of death from cardiovascular disease and ischemic heart disease, compared to the appendicitis cases.
More studies are needed to increase our understanding of long-term effects of heat-related illness. The severity of illness, the duration of exposures, and the etiology and prevention of heat cramps are just a few of many factors that may have an effect on a worker’s chronic condition.
5| Measurement of Heat Stress
Heat stress is the net heat load to which a worker is exposed from the combined contributions of metabolic heat, environmental factors, and clothing worn which results in an increase in heat storage in the body. The heat load experienced by the worker provokes a physiological response (heat strain) which functions to increase heat loss from the body in order to maintain a stable body temperature. This is not always successful and, when unsuccessful, may result in heat injury and death. The environmental factors of heat stress are temperature and movement of air, water vapor pressure, and radiant heat. Physical work contributes to total heat stress of a job by producing metabolic heat in the body in proportion to the work intensity. The amount, thermal characteristics, and type of clothing worn also contribute by altering the rate of heat exchange between the skin and the air [OSHA 1999].
Heat stress may be assessed by measuring the climatic and physical factors of the environment and then evaluating their effects on the human body via an appropriate heat stress index. This chapter presents information on (1) measurement of environmental factors, (2) prediction of meteorological factors from National Weather Service data, and (3) measurement of metabolic heat.
5.1 Environmental Factors
The environmental factors of concern in occupational heat stress are (1) air (dry bulb) temperature; (2) humidity or, more precisely, water vapor pressure; (3) air velocity; and (4) radiation (solar and infrared). One can measure “heat exposure,” which is the amount of heat experienced by the worker. Heat stress is the thermal environment, including radiant temperature, air temperature, humidity, activity, and other factors imposed on a person that can increase body heat storage. Heat strain is the physiological response to the heat stress that attempts to transfer the stored heat back to the environment [Parsons 2003].
5.1.1 Air (Dry Bulb) Temperature
The dry bulb temperature (ta) is the simplest to measure of the climatic factors. It is the temperature of the ambient air, measured with a thermometer. Temperature units are in degrees Celsius [°C = (°F − 32) × 5/9]. The primary types of thermometers used for measuring dry bulb temperature are (a) liquid-in-glass thermometers, (b) thermocouples, and (c) resistance thermometers (thermistors). These thermometers differ in the nature, properties, characteristics, and materials of their sensing elements.
General precautions must be considered in using any thermometer [Ramsey and Beshir 2003]:
- The temperature to be measured must be within the measuring range of the thermometer.
- The time allowed for measurement must be greater than the time required for thermometer stabilization.
- The sensing element must be in contact with or as close as possible to the area of thermal interest.
- Under radiant conditions (i.e., in sunlight or where the temperature of the surrounding surfaces is different from the air temperature), the sensing element should be shielded.
5.1.1.1 Liquid-in-Glass Thermometers
Although a thermometer is any instrument for measuring temperature, this term is commonly identified with the liquid-in-glass thermometer, which is the simplest, most familiar, and most widely used. Historically, mercury and alcohol are the more commonly used liquids. Mercury-in-glass thermometers are preferred under hot conditions, whereas alcohol-in-glass thermometers are preferred under cold conditions, since the freezing point of mercury is -40°C (-40°F) and that of alcohol is -114°C (-173.6°F). Because of the environmental hazards of mercury, these thermometers are not generally available to the public (but are available for research). Therefore, other liquids such as alcohol are used in most generally available thermometers. Thermometers used for measuring dry bulb temperature must be total immersion types. These thermometers are calibrated by total immersion in a thermostatically controlled medium, and their calibration scale depends on the coefficients of expansion of both the glass and the liquid. Only thermometers with the graduations marked on the stem should be used. These thermometers are simple to use but may be fragile and can be affected by radiation.
5.1.1.2 Thermocouples
A thermocouple consists of two wires of different metals connected together at both ends by soldering, welding, or merely twisting to form a pair of junctions. One junction is kept at a constant reference temperature (e.g., usually at 0°C, or 32°F) by immersion in an ice bath. The second junction is exposed to the measured temperature. Due to the difference in electrochemical properties of the two metals, an electromotive force (emf), or voltage, is created, whose potential is a function of the temperature difference between the two junctions. With use of a millivolt meter or a potentiometer to measure the existing emf or the induced electric current, respectively, the temperature of the second junction can be determined from an appropriate calibration table or curve. Copper (a metal) and constantan (a copper-nickel alloy) are the most commonly used to form the thermocouple. Thermocouples are less affected by radiation, are highly accurate, have a fast response, and may be used for remote measurements.
5.1.1.3 Resistance Thermometers
A resistance thermometer, or thermistor, uses a metal wire (i.e., a resistor) as its sensing element; the resistance of the sensing element increases as the temperature increases. The resistance of the sensor element is determined with a Wheatstone bridge and/or a galvanometer, enabling the temperature to be determined from an appropriate calibration table or curve. In some cases, the thermistors are calibrated to give a direct temperature reading. Thermistors are also less sensitive to radiation, but probes may require individual calibration.
5.1.1.4 Bimetallic Thermometers
Bimetallic thermometers are composed of two strips of different metals connected to each other at one end. They operate according to each metal’s coefficient of expansion; when the strips are heated or cooled, they change length by a differing amount, which produces a movement in an indicator calibrated to temperature. These thermometers are frequently used in thermostats and appliances.
5.1.2 Humidity
Humidity, the amount of water vapor within a given space, is commonly measured as the relative humidity (RH), that is, the percentage of moisture in the air relative to the amount it could hold if saturated at the same temperature. Humidity is important as a temperature-dependent expression of the actual water vapor pressure, which is the key climatic factor affecting heat exchange between the body and the environment by evaporation. The higher the water vapor pressure, the lower the evaporative heat loss will be.
A hygrometer, or psychrometer, measures humidity; however, the term is commonly used for those instruments which yield a direct reading of RH. Hygrometers utilizing hair or other organic material are rugged, simple, and inexpensive, but they have low sensitivity, especially at temperatures above 50°C (122°F) and RH below 20%.
5.1.2.1 Water Vapor Pressure
Vapor pressure (Pa) is the pressure at which a vapor can accumulate above its liquid if the vapor is kept in confinement and the temperature is held constant. Vapor pressure is generally expressed in millimeters of mercury (mmHg). However, International System of Units (SI) units for water vapor pressure are kilopascals (kPa). To convert mmHg to kPa, multiply by 7.5 (760/101.325). To calculate heat loss by evaporation of sweat, the ambient water vapor pressure must be used. The lower the ambient water vapor pressure, the higher the rate of evaporative heat loss will be.
Water vapor pressure is most commonly determined from a psychrometric chart. The psychrometric chart graphically represents the relationships among the dry bulb temperature (ta), wet bulb temperature (twb), dew point temperature (tdp), RH, and vapor pressure (Pa). By knowing any two of these five climatic factors, one can obtain the other three from the psychrometric chart.
5.1.2.2 Natural Wet Bulb Temperature
The natural wet bulb temperature (tnwb) is the temperature measured by a thermometer in which the sensor is covered by a wetted cotton wick and is exposed only to the natural prevailing air movement.
The tnwb should be measured only with a liquid- in-glass partial immersion thermometer, which is calibrated by immersing only its bulb in a thermostatically controlled medium. If a total immersion thermometer is used, the measurements must be corrected by applying a correction factor [Benedict 1977]. Accurate measurements of tnwb require using a clean wick, distilled water, and proper shielding to prevent radiant heat gain.
5.1.2.3 Psychrometric Wet Bulb Temperature
The psychrometric wet bulb temperature (tpwb) is obtained when the wetted wick covering the sensor is exposed to a high forced-air movement. The tpwb is commonly measured with a psychrometer, which consists of two mercury-in-glass thermometers mounted alongside each other on the frame of the psychrometer. One thermometer is used to measure the tpwb by covering its bulb with a clean cotton wick wetted with water and the second measures the dry bulb temperature (ta). The air movement is obtained manually with a sling psychrometer or mechanically with a motor-driven psychrometer. The sling psychrometer is usually whirled by a handle, which is jointed to the frame, for about 1 minute. A motor-driven psychrometer uses a battery or spring-operated fan to pull air across the wick. When no temperature change occurs between two repeated readings, measurement of tpwb is taken. Psychrometers are simple, more precise, and faster responding than hygrometers; however, they cannot be used under temperatures near or below the freezing point of water (humidity is usually 100% and water vapor pressure is about 3 mmHg [0.4 kPa]).
5.1.2.4 Dew Point Temperature
Dew point temperature (tdp) is the temperature at which the condensation of water vapor in air begins for a given state of humidity and pressure as the vapor temperature is reduced. The dew point hygrometer measures the dew point temperature by means of cooling a highly polished surface exposed to the atmosphere and observing the temperature at which condensation starts. Dew point hygrometers are more precise than other hygrometers and are useful in laboratory measurements; however, they are more expensive and less rugged than the other humidity-measuring instruments and generally require an electric power source.
5.1.3 Air Velocity
Wind, whether generated by body movements or air movement (Va), is the rate in feet per minute (fpm) or meters per second (m·s-1) at which the air moves. Wind is important in heat exchange between the human body and the environment because of its role in convective and evaporative heat transfer.
Wind velocity is measured with an anemometer. The two major types are vane anemometers (swinging and rotating) and thermoanemometers. Accurate wind velocity contour maps in a work area are very difficult to develop because of the large variability in air movement over time and within space. In this case, the thermoanemometers are quite reliable and are sensitive to 0.05 m·s-1 (10 fpm) but are not very sensitive to wind direction.
If an anemometer is not available for accurate air velocity measurement, then air velocity can be estimated as follows [Ramsey and Beshir 2003]:
Air movement | Vam·s-1 | Vafpm |
---|---|---|
No sensation of air movement (e.g., closed room without any air source) | Va<0.2 | 39 |
Sensing light breezes (e.g., slight perception of air movement) | 0.2<Va<1.0 | 39–197 |
Sensing moderate breezes (e.g., few meters away from a fan; defnite perception of air movement; air causing movement of hair and paper) | 1.0<Va<1.5 | 197–235 |
Sensing heavy breezes (e.g., located close to a fan; air causing marked movement of clothing) | Va>1.5 | >235 |
5.1.3.1 Vane Anemometers (swing and cup)
Vane anemometers are often used for weather forecasting and reporting wind speed. The two major types are the propeller (or rotating) vane and the deflecting (or swinging) vane anemometers. The propeller (or rotating) vane anemometer consists of a light, rotating, wind-driven wheel enclosed in a ring. It indicates the number of revolutions of the wheel or the linear distance in meters or feet. Another type of rotating anemometer consists of three or four hemispherical cups mounted radially from a vertical shaft. Wind from any direction causes the cups to rotate the shaft, and wind speed is determined from the shaft speed [ASHRAE 1981a]. The swinging anemometer consists of a vane enclosed in a case, which has an inlet and an outlet air opening. The vane is placed in the pathway of the air and the movement of the air causes the vane to deflect. This deflection can be translated to a direct readout of the wind velocity by means of a gear train. Rotating vane anemometers are more accurate than swinging vane anemometers.
5.1.3.2 Thermoanemometers
Air velocity is determined with thermoanemometers by measuring the cooling effect of air movement on a heated element. Two types are hot-wire anemometers, which use resistance thermometers, and heated thermocouple anemometers. Two measurement techniques are used: (1) bring the resistance (voltage) of a hot-wire anemometer or the electromotive force (emf) of a heated thermocouple to a specified value, measure the current required to maintain this value, and then determine the wind velocity from a calibration chart; or (2) heat the thermometer (usually by applying a specific electric current) and then determine the air velocity from a direct reading or a calibration chart relating air velocity to the wire resistance of the hot-wire anemometer or to the emf of the heated thermocouple anemometer.
5.1.4 Heat Radiation
Radiant heat sources can be classified as artificial (e.g., infrared radiation in the iron and steel industries, the glass industry, and foundries) or natural (i.e., solar radiation). Instruments that are used for measuring radiation (black globe thermometers or radiometers) have different characteristics from pyrheliometers or pyranometers, which are used to measure solar radiation. However, the black globe thermometer is the most commonly used instrument for measuring the thermal load of solar and infrared radiation on man.
5.1.4.1 Artificial (Non-solar or Occupational) Radiation
(1) Black Globe Thermometers
In 1932, Vernon developed the black globe thermometer to measure radiant heat. The thermometer consists of a 15-centimeter (6-inch) hollow copper sphere (a globe) painted a matte black to absorb the incident infrared radiation (0.95 emissivity) and a sensor (thermistor, thermocouple, or mercury-in-glass partial immersion thermometer), with its sensing element placed in the center of the globe. The Vernon globe thermometer is the most commonly used device for evaluating occupational radiant heat, and it is recommended by NIOSH for measuring the black globe temperature (tg) [NIOSH 1972]; it is sometimes called the standard 6-inch black globe.
Black globe thermometers exchange heat with the environment by radiation and convection. The temperature stabilizes when the heat exchange by radiation is equivalent to the heat exchange by convection. Both the thermometer stabilization time and the conversion of globe temperature to mean radiant temperature are functions of the globe size [Kuehn 1973]. The standard 6-inch globe requires a period of 15 to 20 minutes to stabilize, whereas small black globe thermometers of 4.2 centimeters (1.65 inches) in diameter, which are commercially available, require about 5 minutes to stabilize [Kuehn and Machattie 1975]. While the Vernon 6 inch (150 mm) black globe it is still used and is considered the standard of the industry (ISO 7243 2008), smaller, hand-held, WBGT units are available equipped with black globes < 2 inches in diameter. However, whether one uses a 6 inch black globe or a hand held 2 inch black globe, the principle is the same [Parsons 2003; McArdle et al. 2010b].
The tg is used to calculate the Mean Radiant Temperature (MRT). The MRT is defined as the temperature of a “black enclosure of uniform wall temperature which would provide the same radiant heat loss or gain as the non-uniform radiant environment being measured.” The MRT for a standard 6-inch black globe can be determined from the following equation:
MRT = tg + (1.8 Va0.5)(tg − ta)
where
MRT = Mean Radiant Temperature (°C)
tg = black globe temperature (°C)
ta = air temperature (°C)
Va = air velocity (m·s-1)
(2) Radiometers
A radiometer is an instrument for measuring infrared radiation. Some radiometers, such as infrared pyrometers, use the measured radiant energy to indicate the surface temperature of the radiant source by measuring radiation emitted from a so-called “black body radiator” or object radiating heat. Surface temperatures ranging from -30° to 3000°C (-22°F to 5432°F) can be measured with an infrared pyrometer. Modern IR pyrometers are hand-held devices that are readily used in an occupational setting [Åstrand et al. 2003].
The net radiometer consists of a thermopile with the sensitive elements exposed on the two opposite faces of a blackened disc. It has been used to measure the radiant energy balance of human subjects [Cena et al. 1981]. A variety of radiometers has been used to measure radiant flux [Gagge 1970]. Radiometers are not commonly used in occupational radiant heat measurements, however. They are used most commonly in laboratories, or for measuring surface temperature and direct radiant energy from the sun falling on the surface of the earth. In addition, radiometers are used to measure skin temperature. The radiometer (skin sensor) is attached to the skin and records changes in skin temperature during exposure to hot environments or during exercise (usually in a laboratory setting) [Åstrand et al. 2003].
5.1.4.2 Natural (Solar) Radiation
Solar radiation can be classified as direct, diffuse, or reflected. Direct solar radiation comes from the solid angle of the sun’s disc and implies no barrier between the sun and the worker. Diffuse solar radiation (sky radiation) is the scattered and reflected solar radiation coming from the whole hemisphere after shading the solid angle of the sun’s disc. Reflected solar radiation is the solar radiation reflected from the ground or water. The total solar heat load is the sum of direct, diffuse and reflected solar radiation, as modified by clothing worn and position of the body relative to the solar radiation [Roller and Goldman 1967].
(1) Pyrheliometers
Direct solar radiation is measured with a pyrheliometer. A pyrheliometer consists of a tube that can be directed at the sun’s disc and a thermal sensor. Generally, a pyrheliometer with a thermopile as sensor and a view angle of 5.7° is recommended [Allen et al. 1976; Garg 1982]. Two different pyrheliometers are widely used: the Angstrom compensation pyrheliometer and the Smithsonian silver disc pyrheliometer, each of which uses a slightly different scale factor.
(2) Pyranometers
Diffuse and total solar radiations can be measured with a pyranometer. For measuring diffuse radiation, the pyranometer is fitted with a disc or a shading ring to prevent direct solar radiation from reaching the sensor. The receiver usually takes a hemispherical dome shape to provide a 180° view angle for total sun and sky radiation. It is used in an inverted position to measure reflected radiation. The thermal sensor may be a thermopile, a silicon cell, or a bimetallic strip. Pyranometers can be used for measuring solar or other radiation between 0.35 and 2.5 micrometers (μm), which includes the ultraviolet, visible, and infrared range. Additional descriptions of solar radiation measurement can be found elsewhere [Duffie and Beckman 1980; Garg 1982; Chang and Ge 1983].
Pyrheliometers and pyranometers are the standard means to measure radiant energy (ISO 9060 Solar energy—Specification and classification of instruments for measuring hemispherical solar and direct solar radiation [1990]). However, a more practical and complete way to measure heat stress in an occupational setting is to use a WBGT. Many of the WBGT devices are hand held and are sufficiently accurate for most occupational settings. Unlike pyrheliometers and pyranometers, WBGT takes into account not only the radiant heat but also humidity and dry air temperature thus providing a more comprehensive measure of all the components of environmental heat stress.
5.1.5 Psychrometric Chart
The psychrometric chart is a graphical representation of the relationships among dry bulb temperature, wet bulb temperature, RH, vapor pressure, and dew point temperature. If any two of these variables are known, any of the others can be determined from the psychrometric chart. Figure 5-1 depicts a standard psychrometric chart [ISO 1993]. Note that when RH equals 100%, dry bulb, wet bulb, and dew point temperature are equal. Psychrometric charts are valuable tools for assessing the thermal environment indoors where there is negligible solar or radiant heat exposure.
Figure 5-1. The psychrometric chart (adapted from ISO [1993] and Coolerado [2012])
5.2 Prediction of Meteorological Factors from the National Weather Service Data
The National Oceanic and Atmospheric Administration’s National Weather Service provides daily environmental measurements, which can be a useful supplement to the climatic factors measured at a worksite. The National Weather Service data include timely observations on air temperature, humidity, wind speed, dew point, and visibility. These data can be used for approximate assessment of the worksite environmental heat load for outdoor jobs or for some indoor jobs where air conditioning is not in use. Atmospheric pressure data can also be used for both indoor and outdoor jobs. The actual and projected data may be helpful in predicting local WBGT [Bernard and Barrow 2013]. In addition, the National Weather Service may issue specific advisories during extreme heat, based on the heat index. The heat index incorporates temperature with RH to estimate the “feels like” temperature [Golden et al. 2008]. A recent study found that 86% of heat injuries were associated with a heat index range of 32.2°C to 40°C (90°F to 104°F) [Armed Forces Health Surveillance 2011]. For additional information on the heat index, see Appendix C.
National Weather Service data have also been used in studies of mortality due to heat-aggravated illness resulting from heat waves in the United States [Semenza et al. 1996; Curriero et al. 2002; Knowlton et al. 2007; Golden et al. 2008]. However, attributing heat waves and extreme heat events (EHE) to related health impacts can be a difficult task. Heat waves are often referred to as silent killers because unlike with other natural disasters such as hurricanes, they do not leave an obvious trail of destruction [Luber and McGeehin 2008]. Despite this, extreme heat is responsible for nearly the same or greater number of deaths in the United States as lightening, storms, floods, and earth movements (e.g., landslides and earthquakes) combined [Berko et al. 2014; Thacker et al. 2008]. Heat-related illnesses and deaths estimates due to a heat wave are often misclassified, unrecognized, or not reported at all [Luber and McGeehin 2008].
Continuous monitoring of the environmental factors at the worksite provides information on the level of heat exposure at the time the measurements are made. Such data are useful for developing heat stress engineering controls. However, in order to have established work practices in place when needed, it is desirable to predict the anticipated level of heat stress for a day or more in advance. A methodology has been developed based on the psychrometric wet bulb for calculating the WBGT at the worksite from the National Weather Service meteorologic data. The data upon which the method is based were derived from simultaneous measurements of the thermal environment in 15 representative worksites, from outside the worksites, and from the closest National Weather Service station. The empirical relationships between the inside and outside data were established. From these empirical relationships, it is possible to predict worksite WBGT, effective temperature (ET), or corrected effective temperature (CET) values from weather forecasts or local meteorologic measurements. To apply the predictions model, it is first necessary to perform a short environmental study at each worksite to establish the differences in inside and outside values and to determine the regression constants that are unique to each workplace, perhaps because of the differences in actual worksite air motion compared to the constant high air motion associated with the use of the ventilated wet bulb thermometer [Mutchler et al. 1976]. Another example involves heat stress evaluation at an aluminum smelter, where targeted environmental measurements were estimated based on National Weather Service data, a task analysis was performed, and heat stress was analyzed on the basis of these elements [Logan and Bernard 1999].
5.3 Metabolic Heat
The total heat load imposed on the human body is the aggregate of environmental and physical work factors. The energy cost of an activity, as measured by the metabolic heat (M), is a major element in the heat-exchange balance between the human body and the environment. The M value can be measured or estimated. The energy cost of an activity is made up of two parts: the energy expended in doing the work and the energy transformed into heat. On the average, muscles may reach 20% efficiency in performing heavy physical work. However, unless external physical work is conducted, the body heat load is approximately equal to the total metabolic energy turnover. For practical purposes, M is equated with total energy turnover.
5.3.1 Measurements of Metabolic Heat
5.3.1.1 Measurement of Metabolic Heat by Direct Calorimetry
To determine the worker’s heat production by direct calorimetry, the subject is placed in a calorimeter, an enclosed chamber surrounded by circulating water; the increase in the temperature of the circulating water is used to determine the amount of heat liberated from the human body. The direct procedure has limited practical use in occupational heat stress studies, because the procedure is difficult and time consuming and the equipment and chambers are expensive [Banister and Brown 1968].
5.3.1.2 Measurements of Metabolic Heat by Indirect Calorimetry
Primary methods of measurements of metabolic heat by indirect calorimetry are based on measuring oxygen consumption. Each liter of oxygen consumed results in the production of approximately 4.8 kcal (5.6 W) of metabolic heat. Indirect calorimetry uses either the closed circuit or the open circuit procedure. An even more indirect procedure for measuring metabolic heat is based on the linear relationship between HR and oxygen consumption. The linearity, however, usually holds only at submaximal HRs because, on approaching the maximum, the pulse rate begins to level off while the oxygen intake continues to rise. The linearity also holds only on an individual basis because of the large inter-individual differences in responses [Karpovich and Sinning 1971; Berger 1982].
(1) Closed Circuit
In the closed circuit procedure, the subject inhales from a spirometer and the expired air returns to the spirometer after passing through carbon dioxide and water vapor absorbents. The depletion in the amount of oxygen in the spirometer represents the oxygen consumed by the subject. The development of computerized techniques, however, has led to revisions of the classic procedures so that equipment and the evaluation can be controlled by a computer, which results in prompt, precise, and simultaneous measurement of the significant variables [Stegman 1981].
(2) Open Circuit
Historically, in the open circuit procedure, the worker breathes atmospheric air and the exhaled air is collected in a large container, that is, a Douglas bag or meteorological balloon. The volume of the expired air can be accurately measured with a calibrated gasometer. The concentration of oxygen in the expired air can be measured by chemical or electronic methods. The oxygen and carbon dioxide in atmospheric air usually average 20.90% and 0.03%, respectively, or they can be measured so that the amount of oxygen consumed and the metabolic heat production for the performed activities can be determined.
Another open circuit procedure, the Max Planck respiration gasometer, eliminates the need for an expired air collection bag and a calibrated gasometer [Stegman 1981]. The subject breathes atmospheric air and exhales into the gasometer, where the volume and temperature of the expired air are immediately measured. An aliquot sample of the expired air is collected in a rubber bladder for later analysis for oxygen and carbon dioxide concentrations. Both the Douglas bag and the respiration gasometer are portable and thus appropriate for collecting expired air of workers at different industrial or laboratory sites [Stegman 1981].
Many new technologies for measuring metabolic rates in humans are now available. These new techniques involve a metabolic cart that samples breathing air on a breath-by-breath basis and calculates oxygen consumption (·V.O2 max), carbon dioxide production (·V.CO2), minute ventilation (·V.E), respiratory exchange ratio (RER), etc. Wearable metabolic carts also can make these measurements [McArdle et al. 2010a]. Nearly every modern exercise physiology laboratory employs the metabolic cart while conducting its research (ISO 8996: Ergonomics of the thermal environment—Determination of metabolic rate [2004]).
5.3.2 Estimation of Metabolic Heat
The procedures for direct or indirect measurement of metabolic heat are limited to relatively short duration activities and require equipment for collecting and measuring the volume of the expired air and for measuring the oxygen and carbon dioxide concentrations. Alternatively, although they are less accurate and reproducible, metabolic heat estimates on the basis of tables of energy expenditure or task analysis can be applied for short and long-duration activities and require no special equipment. However, the accuracy of the estimates made by a trained observer may vary by about ±10% to 15%. A training program consisting of supervised practice in using the tables of energy expenditure in a workplace situation will usually result in an increase in accuracy of the estimates of metabolic heat production [AIHA 1971; Garg et al. 1978].
5.3.2.1 Tables of Energy Expenditures
Estimates of metabolic heat for use in assessing muscular workload and human heat regulation are commonly obtained from tabulated descriptions of energy cost for typical work tasks and activities [Smith and Ramsey 1980; ACGIH 2014]. Errors in estimating metabolic rate from energy expenditure tables are reported to be as high as 30% [ISO 1990]. The ISO [1990] recommends that the metabolic rate be estimated by adding the following values: (1) basal metabolic rate, (2) metabolic rate for body position or body motion, (3) metabolic rate for type of work, and (4) metabolic rate related to work speed. The basal metabolic rate averages 44 and 41 W·m-2 for the “standard” man and woman, respectively. Metabolic rate values for body position, and body motion, type of work, and those related to work speed are provided [ISO 1990].
5.3.2.2 Task Analysis
In order to evaluate the average energy requirements over an extended period of time for a task, including both work and rest activities, it is necessary to divide the task into its basic activities and subactivities. The metabolic heat of each activity or subactivity is then measured or estimated and a time-weighted average for the energy required for the task can be obtained. It is common in such analyses to estimate the metabolic rate for the different activities by utilizing tabulated energy values from tables (see Table 5-1) that specify incremental metabolic heat resulting from the movement of different body parts (e.g., arm work, leg work, standing, and walking) [McArdle et al. 1996b]. The metabolic heat of the activity can then be estimated by summing the component M values based on the actual body movements.
6| Control of Heat Stress
According to the heat balance equation [S = (M − W) ± C ± R ± K – E] described in Section 3.1, heat stress can be reduced only by modifying one or more of the following factors: metabolic heat production, heat exchange by convection, heat exchange by radiation, or heat exchange by evaporation. Environmental heat load (C, R, and E) can be modified by engineering controls (e.g., ventilation, air conditioning, screening, insulation, and modification of process or operation) and protective clothing and equipment, whereas metabolic heat production can be modified by work practices and application of labor-reducing devices. Each of these alternative control strategies will be discussed separately. Actions that can be taken to control heat stress and strain are listed in Table 6-1 [Belding 1973].
6.1 Engineering Controls
The environmental factors that can be modified by engineering procedures are those involved in convective, radiative, and evaporative heat exchange.
6.1.1 Convective Heat Control
As discussed earlier, the environmental variables concerned with convective heat exchange between the worker and the ambient environment are dry bulb air temperature (ta) and the speed of air movement (Va). When air temperature is higher than the mean skin temperature (tsk of 35°C or 95°F), heat is gained by convection. The rate of heat gain is dependent on temperature differential (ta − tsk) and air velocity (Va), where if ta is below tsk, then heat is lost from the body; the rate of loss is dependent on ta − tsk and air velocity.
Engineering approaches to enhancing convective heat exchange are limited to modifying air temperature and air movement. When ta is less than tsk, increasing air movement across the skin by increasing either general or local ventilation will increase the rate of body heat loss. When ta exceeds tsk (convective heat gain), ta should be reduced by bringing in cooler outside air or by evaporative or refrigerative cooling of the air. In addition, as long as ta exceeds ¯tsktsk, air speed should be reduced to levels that will still permit sweat to evaporate freely but will reduce convective heat gain (see Table 6-1). The effect of air speed on convective heat exchange is a function of air speed. Spot cooling (ta less than ¯tsk) of the individual worker can be an effective approach to controlling convective heat exchange, especially in large workshops where the cost of cooling the entire space would be prohibitive. However, spot coolers or blowers may interfere with the ventilating systems required to control toxic chemical agents.
6.1.2 Radiant Heat Control
Radiant heat exchange between the worker and hot equipment, processes, and walls that surround the worker is a fourth power function of the difference between skin temperature (¯tsk) and the temperature of hot objects that are in direct, unobstructed line to the worker (tr). The only engineering approaches to controlling radiant heat gain are to reduce tr or to shield the worker from the radiant heat source.
To reduce tr would require (1) lowering the process temperature, which is usually not compatible with the temperature requirements of the manufacturing processes; (2) relocating, insulating, or cooling the heat source; (3) placing line-of-sight radiant reflective shielding between the heat source and the worker; or (4) changing the emissivity of the hot surface by coating the material. Of the alternatives, radiant reflective shielding is generally the easiest to install and the least expensive. Radiant reflective shielding can reduce the radiant heat load by as much as 80% to 85%. Some ingenuity may be required in placing the shielding so that it does not interfere with the work. Remotely operated tongs, metal chain screens, or air- or hydraulically activated doors that are opened only as needed are some of the possibilities.
6.1.3 Evaporative Heat Control
Heat is lost from the body when sweat evaporates from the skin surface. The rate and amount of evaporation are functions of the speed of air movement over the skin and the difference between the water vapor pressure of the air (pa) at ambient temperature and the water vapor pressure of the wetted skin (with an assumed skin temperature of 34°C–35°C [93.2°F–95°F]). At any air-to-skin vapor pressure gradient, the evaporation increases as a 0.6 root function of increased air movement. Evaporative heat loss at low air velocities can be greatly increased by improving ventilation (increasing air velocity). At high air velocities (2.5 m·s-1 or 500 fpm), an additional increase will be ineffective, except when the clothing worn interferes with air movement over the skin.
Engineering control of evaporative cooling can be accomplished in two ways: (1) increase air movement or (2) decrease ambient water vapor pressure. Of these, increasing air movement by the use of fans or blowers is often the simplest and usually the cheapest approach to increasing the rate of evaporative heat loss. Ambient water vapor pressure reduction usually requires air-conditioning equipment (cooling compressors). In some cases, the installation of air conditioning, particularly spot air conditioning, may be less expensive than the installation of increased ventilation because of the lower airflow involved. The vapor pressure of the worksite air is usually at least equal to that of the outside ambient air, except when all incoming and recirculated air is humidity controlled by absorbing or condensing the moisture from the air (i.e., by air conditioning). In addition to the ambient air as a source of water vapor, water vapor may be added from the manufacturing processes as steam, leaks from steam valves and steam lines, and evaporation of water from wet floors. Eliminating these additional sources of water vapor can help reduce the overall vapor pressure in the air and thereby increase evaporative heat loss by facilitating the rate of evaporation of sweat from the skin [Dasler 1977].
6.2 Administrative Controls
The job risk factors for occupational heat stress are thermal environment, work demands, and clothing requirements. These are reflected in occupational exposure limits (OELs) traditionally based on WBGT, such as NIOSH RELs and ACGIH TLVs®, and in ISO 7243. Many workers spend some part of their working day in a hot environment where the temperature is above the OELs. Strategies to reduce the effects of heat in the workplace include primarily eliminating the heat with engineering controls, although in some situations administrative controls and PPE will be necessary.
In some situations, it may be technologically impossible or impractical to completely control heat stress by the application of engineering controls; the level of environmental heat stress may be unpredictable and variable (as in seasonal heat waves), and exposure time may vary with the task and with unforeseen critical events. When applying engineering controls for heat stress is not practical or sufficient, other solutions must be sought to keep the worker’s total heat stress level within limits that will not be associated with an increased risk of heat-related illnesses.
Administrative controls consist of mainly five strategies: (1) limiting or modifying the duration of exposure time; (2) reducing the metabolic component of the total heat load; (3) enhancing the heat tolerance of the workers by, for example, heat acclimatization and physical conditioning; (4) training the workers in safety and health procedures for work in hot environments; and (5) medical screening of workers to be aware of which individuals have low heat tolerance and/or low physical fitness.
6.2.1 Limiting Exposure Time and/or Temperature
There are several ways to control the daily length of time and temperature to which a worker is exposed in heat stress conditions [OSHA-NIOSH 2011].
- When possible, schedule hot jobs for the cooler part of the day (early morning, late afternoon, or night shift) and/or schedule hot jobs on alternate rather than successive days.
- Schedule routine maintenance and repair work in hot areas for the cooler seasons of the year.
- Redesign the work to increase distance from radiant heat sources.
- Alter the work/rest schedule to permit more rest time (see examples in Tables 6-2 and 6-3).
- Provide cool areas (e.g., air-conditioned or shaded) for rest and recovery.
- Add extra personnel to reduce exposure time for each member of the crew.
- Permit work interruption when a worker feels heat discomfort.
- Increase workers’ water intake on the job.
- Adjust schedule, when possible, so that hot operations are not performed at the same time and place as other operations that require the presence of workers, such as maintenance and cleanup while tapping a furnace.
- Utilize the Thermal Work Limit guide as the limiting (or maximum) sustainable metabolic rate that well-hydrated, acclimatized individuals can maintain in a specific thermal environment, within a safe deep body core temperature (< 38.2°C or 100.8°F) and sweat rate (< 1.2 kg or 2.6 lb per hour). The index is designed for self-paced workers and does not rely on estimation of actual metabolic rates, a process that is difficult and subject to considerable error. However, the maximum metabolic rate that can be maintained under these circumstances is limited by the workers overall fitness, not simply level of hydration and acclimatization to the heat. The Thermal Work Limit is therefore of limited use because of this.
6.2.2 Reducing Metabolic Heat Load
In most work situations, metabolic heat is not the major part of the total heat load. However, because it represents an extra load on the circulatory system, it can be a critical component in high heat exposures. Heavy and very heavy metabolic rates require substantial rest periods. For some examples of work/rest schedules, see Tables 6-2 and 6-3. Metabolic heat production can be reduced—but usually by not more than 200 kcal·h-1(800 Btu·h-1)—by these strategies:
- Mechanization of the physical components of the job
- Reduction of work time (reduce work day, increase rest time, restrict double- shifting) and planned times (e.g., U.S. Navy Physiological Heat Exposure Limit [PHEL] times, Electric Power Research Institute [EPRI] action times, University of South Florida (USF) WBGT–based Safe Exposure Times [Bernard & Ashley 2009], Predicted Heat Strain [ISO 7933])
- Increase of the work force.
6.2.3 Enhancing Tolerance to Heat
Stimulating the human heat-adaptive mechanisms can significantly increase the capacity to tolerate work in heat. However, the ability of people to adapt to heat varies widely, which must be kept in mind when considering any group of workers.
A properly designed and applied heat-acclimatization program will increase the ability of workers to work at a hot job and will decrease the risk for heat-related illnesses and unsafe acts. Heat acclimatization can usually be induced in 7 to 14 days of exposure at the hot job [DOD 2003; Navy Environmental Health Center 2007; ACGIH 2014]. For workers who have had previous experience with the job, the acclimatization regimen should be no more than 50% of the usual duration of work in the hot environment on day 1, 60% on day 2, 80% on day 3, and 100% on day 4. For new workers, the schedule should be no more than 20% of the usual duration of work in the hot environment on day 1, increasing by no more than 20% each day.
Being physically fit for the job will not replace heat acclimatization but can enhance heat tolerance for both heat-acclimatized and nonacclimatized workers [Pandolf et al. 1977; DOD 2003; Yeargin et al. 2006; Navy Environmental Health Center 2007]. The time required for non–physically fit individuals to develop acclimatization is about 50% greater than for the physically fit. For more information on acclimatization, see Table 4-1.
To ensure that water lost in the sweat and urine is replaced (at least hourly) during the work day, an adequate water supply and intake are essential for heat tolerance and prevention of heat-related illnesses.
Electrolyte balance in the body fluids must be maintained to help prevent heat-related illnesses. For unacclimatized workers who may be on a salt-restricted diet, additional salting of the food, with the concurrence of the responsible healthcare provider, during the first two days of heat exposure, may be needed to replace the salt lost in the sweat [Lind 1976; DOD 2003]. The heat-acclimatized worker loses relatively little salt in sweat and therefore usually does not need salt supplementation.
6.2.4 Health and Safety Training
A heat stress training program should be in place for all who work in hot environments and their supervisors. Workers and supervisors should be trained about the prevention and first aid of heat-related illness before they begin work in a hot environment and before heat index levels go up. Heat prevention training should be reinforced on hot days. Prevention of heat-related illnesses depends on early recognition of the signs and symptoms of impending heat-related illness and initiation of first aid and corrective procedures at the earliest possible moment.
Employers should provide a heat stress training program that effectively trains all workers and supervisors about the following:
- Recognition of the signs and symptoms of the various types of heat-related illnesses—such as heat cramps, heat exhaustion, heat rash, and heat stroke—and in administration of first aid (see Table 4-3).
- The causes of heat-related illnesses and the personal care procedures that will minimize the risk of their occurrence, such as drinking enough water and monitoring the color and amount of urine output (see Appendix B).
- The proper care and use of heat-protective clothing and equipment and the added heat load caused by exertion, clothing, and personal protective equipment.
- The effects of nonoccupational factors (drugs, alcohol, obesity, etc.) on tolerance to occupational heat stress.
- The importance of acclimatization (see Table 4-1).
- The importance of immediately reporting to the supervisor any symptoms or signs of heat-related illness in themselves or in their coworkers.
- The employer’s procedures for responding to symptoms of possible heat-related illness and for contacting emergency medical services if needed.
In addition to being trained about each of those topics, supervisors should be trained on the following:
- How to implement appropriate acclimatization.
- What procedures to follow when a worker has symptoms consistent with heat-related illness, including emergency response procedures.
- How to monitor weather reports.
- How to respond to hot weather advisories.
- How to monitor and encourage adequate fluid intake and rest breaks.
A buddy system should be initiated, in which workers on hot jobs are taught to recognize the early signs and symptoms of heat-related illness. Each worker and supervisor who has received the instructions is assigned the responsibility for observing, at periodic intervals, one or more fellow workers to determine whether they have any early symptoms of a heat-related illness. Any worker who exhibits signs and symptoms of an impending heat-related illness should be sent to the first-aid station for more complete evaluation and possible initiation of first-aid treatment. Workers on hot jobs where the heat stress exceeds the RAL or REL (for unacclimatized and acclimatized workers, respectively) should be observed by a fellow worker or supervisor.
Additional training and educational materials are available from:
- NIOSH at http://www.cdc.gov/niosh/topics/heatstress/
- OSHA at https://www.osha.gov/SLTC/...
- Cal/OSHA at http://www.dir.ca.gov/DOSH/HeatIllnessInfo.html
6.2.5 Screening for Heat Intolerance
The ability to tolerate heat stress varies widely, even between healthy individuals with similar heat exposure experiences [Shvartz and Benor 1972; Wyndham 1974; Strydom 1975; Khogali 1997; Moran et al. 2007]. Heat intolerance factors in young, active persons may be congenital (e.g., ectodermal dysplasia or chronic idiopathic anhidrosis), functional (e.g., low physical fitness, lack of acclimatization, low work efficiency, or reduced skin area to body mass ratio), or acquired (e.g., sweat gland dysfunction, dehydration, infectious disease, x-ray irradiation, previous heat stroke, large scarred burns, or drugs) [Epstein et al. 1997; Moran et al. 2007]. One way to reduce the risk of heat-related illnesses and disorders within a heat-exposed workforce is to reduce or eliminate the exposure of heat-intolerant individuals to heat stress. The ability to identify heat-intolerant individuals, without resorting to strenuous, time-consuming heat-tolerance tests, is basic to any such screening process.
Data from laboratory and field studies indicate that individuals with low physical work capacity are more likely to develop higher body temperatures than are individuals with high physical work capacity when exposed to equally hard work in high temperatures. In these studies, none of the individuals with a maximum work capacity (·V.O2 max) of at least 2.5 liters of oxygen per minute (L∙min-1) were heat intolerant, but 63% of those with ·V.O2 max below 2.5 L∙min-1 were. It has also been shown that heat-acclimatized individuals with a ·V.O2 max less than 2.5 L∙min-1 had a 5% risk of reaching heat stroke levels of body temperature (40°C, or 104°F), whereas those with a ·V.O2 max above 2.5 L∙min-1 had only a 0.05% risk [Wyndham 1974a; Strydom 1975].
Medical screening for heat intolerance in otherwise healthy individuals should include obtaining a history of any previous incidents of heat-related illness. Workers who have experienced a heat-related illness may be less heat-tolerant [Leithead and Lind 1964; Armstrong et al. 1990]. In a study by Moran et al. [2007], a heat tolerance test (HTT) was evaluated and found to be efficient at differentiating between a temporary and permanent state of heat susceptibility, either of which could occur following exertional heat stroke. The test is described as a 120 minute exposure to 40°C and 40% RH in a climatic chamber while walking on a treadmill. The person being tested wears shorts and a t-shirt and walks at a pace of 5 km·h-1 (3 mph) at a 2% elevation. Rectal temperature and heart rate are continuously monitored. Sweat rate is determined by differences in weight and corrected for fluid intake. At the end of the test, measurements for heat-tolerant individuals will be rectal temperature of 38 ± 0.3°C, heart rate of 120 ± 15 bpm, and sweat rate of 780 ± 160g·h-1. Heat intolerance is determined when the rectal temperature is higher than 38.5°C (101.3°F) or the heart rate exceeds 145 bpm. Larger increases indicate heat intolerance. Moran suggests that the HTT be conducted within 6 to 8 weeks after a heat exhaustion or exertional heat stroke episode and that the test may be repeated 4 to 8 weeks later to evaluate the diagnosis of heat intolerance. However, it should be noted that heart rate varies in populations with differing levels of fitness and age. Therefore, heart rate must be used cautiously to determine heat tolerance.
6.2.6 Heat Alert Program
When heat-related illnesses and disorders occur mainly during heat waves in the summer, a Heat Alert Program (HAP) should be established for preventive purposes. Although such programs differ in detail from one worksite to another, they all use the weather forecast of the National Weather Service. If a heat wave is predicted for the next day or days, a state of Heat Alert is declared to make sure that measures to prevent heat casualties will be strictly observed. Although this sounds quite simple and straightforward, in practical application, it requires the cooperation of the administrative staff, the maintenance and operative workforce, and the medical, industrial hygiene, and/or safety departments. An effective HAP is described below [Dukes-Dobos 1981]. Although this HAP is designed for indoor work settings, many aspects can also be used or modified for outdoor work settings such as in construction or agriculture.
- Each year, early in the spring, establish a Heat Alert Committee, which may consist of a responsible healthcare provider, industrial hygienist or qualified safety and health professional, safety engineer, operation engineer, and manager. Once established, this committee takes care of the following tasks:
- Arrange a training course for all involved in the HAP that provides procedures to follow in the event a Heat Alert is declared; emphasize the prevention and early recognition of heat-related illnesses and first aid procedures when a heat-related illness occurs.
- By memorandum, instruct the supervisors to perform these tasks:
- Reverse winterization of the site, that is, open windows, doors, skylights, and vents according to instructions and if appropriate (e.g., outside temperatures are cooler) for greatest ventilating efficiency at places where high air movement is needed.
- Check drinking fountains, fans, and air conditioners to make sure that they are functional, that the necessary maintenance and repairs are performed, that they are regularly rechecked, and that workers know how to use them.
- Ascertain that, in the medical department, as well as at the job sites, all facilities required to give first aid in cases of heat-related illness are in a state of readiness.
- Establish criteria for the declaration of a Heat Alert. For instance, a Heat Alert would be declared if the area weather forecast for the next day predicts a maximum air temperature of at least 35°C (95°F) or if a maximum temperature of 32°C (90°F) is predicted and is 5°C (9°F) higher than the temperature reached on any of the preceding three days.
- Procedures to be followed during the state of Heat Alert are as follows:
- Postpone tasks that are not urgent (e.g., preventive maintenance involving high activity or heat exposure) until the heat wave is over.
- Increase the number of workers on each team in order to reduce each worker’s heat exposure. Introduce new workers gradually to allow acclimatization (follow heat-acclimatization procedure).
- Increase rest allowances. Let workers recover in air-conditioned rest places.
- Turn off heat sources that are not absolutely necessary.
- Remind workers to drink water in small amounts frequently to prevent excessive dehydration, to weigh themselves before and after the shift, and to be sure to drink enough water to maintain body weight.
- Monitor the environmental heat at the job sites and resting places.
- Check workers’ core temperature during their most severe heat-exposure period.
- Exercise additional caution on the first day of a shift change to make sure that workers are not overexposed to heat, because they may have lost some of their acclimatization over the weekend and during days off.
- Send workers who show signs of a heat disorder, even a minor one, for medical evaluation. Permission of the responsible healthcare provider to return to work must be given in writing.
- Restrict overtime work.
- Suspend piece-rate payment mechanisms.
6.3 Personal Protective Clothing and Auxiliary Body Cooling
When unacceptable levels of heat stress occur, there are generally only four approaches to a solution: (1) modify the work; (2) modify the environment; (3) modify the worker by heat acclimatization; or (4) modify the clothing or equipment. Improving human tolerance requires that the individuals be fully heat acclimated to the level of heat, be trained in the use of and practice in wearing protective clothing, be in good physical condition, and be encouraged to drink as much water as necessary (e.g., 1 cup [8 oz.] of water or other fluids every 15–20 minutes, or see Table 8-1) to compensate for sweat water loss.
The issues of clothing and its effects on heat exchange have been discussed in detail elsewhere in this document (see 3.3 Effects of Clothing on Heat Exchange). Wearing impermeable clothing (e.g., PPE) effectively prevents heat exchange (i.e., conduction, convection, radiation, and sweat evaporation) from the body to the external environment. Moreover, the additional metabolic energy required to wear and work in the clothing produces a greater amount of heat than would occur without the impermeable clothing adding to the heat burden. Unfortunately, the necessity of wearing an impermeable clothing ensemble suggests the need to protect against a significant environmental hazard that could injure or kill the worker. Since the PPE is required to protect against this hazard and yet produces its own heat hazard, there is a need to protect against the heat stress induced by the impermeable garment. The following sections discuss the various personal cooling technology used in conjunction with encapsulating and impermeable PPE to limit the heat stress imposed by these garments.
It may be possible to redesign ventilation systems for occupied spaces to avoid interior humidity and temperature buildup; however, such redesign may not completely solve the heat stress problem. When air temperature is above 35°C (95°F) with an RH of 75% to 85%, or when there is an intense radiant heat source, a suitable (and in some ways more functional) approach is to modify the clothing to include some form of auxiliary body cooling. Even mobile individuals afoot can be provided some form of auxiliary cooling for limited periods of time. A properly designed system will reduce heat stress, conserve large amounts of drinking water that would otherwise be required, and allow unimpaired performance across a wide range of climatic factors. A seated individual will rarely require more than 100 W (86 kcal∙h-1 or 344 Btu∙h-1) of auxiliary cooling, and the most active individuals, not more than 400 W (345 kcal∙h-1 or 1380 Btu∙h-1), unless they work at a level where physical exhaustion would limit the duration of work. Some form of heat-protective clothing or equipment should be provided for exposures at heat stress levels that exceed the RAL or REL (Figures 8-1 and 8-2).
Auxiliary cooling systems can range from simple approaches such as applying frozen materials under the clothing to more complex systems, such as cooled garments; however, cost of logistics and maintenance are considerations of varying magnitude in all of these systems. Four auxiliary cooling approaches have been evaluated: (1) water-cooled garments, (2) air-cooled garments, (3) cooling vests, and (4) wetted overgarments. Each of these auxiliary body cooling approaches might be applied in alleviating risk of severe heat stress in a specific workplace setting [Goldman 1973, 1981].
6.3.1 Water-cooled Garments
Water-cooled garments have been designed and constructed in various forms with significant improvements on both engineering and physiological perspectives. Water-cooled garments provide cooling by means of conductive heat exchange between skin and coolant tubing sewn inside a garment in which a network of tubing is distributed onto either a whole body or limited body regions. Water-cooled garments also require an external device for operation, which may include battery, circulating pump, heat exchanger, fluid container, and control pad. The weight and volume of the operating device may limit a wearer’s movement and impose an extra weight burden; this factor, along with the nature of the work and environmental conditions, will determine the effective use time of the water-cooled garment. In addition, at water temperatures at or below the dew point, condensation around the tubes may increase heat loss from the skin through permeable clothing [Nag et al. 1998].
The range of cooling provided by each of the water-cooled garments versus the cooling water inlet temperature has been studied. The rate of increase in cooling, with decrease in cooling water inlet temperature, is 3.1 W·°C-1 for the water-cooled cap with water-cooled vest, 17.6 W·°C for the short water-cooled undergarment, and 25.8 W·°C-1 for the long water-cooled undergarments. A “comfortable” cooling water inlet temperature of 20°C (68°F) should provide 46 W of cooling with use of the water-cooled cap; 66 W with use of the water-cooled vest; 112 W with use of the water-cooled cap and water-cooled vest together; 264 W with use of the short water-cooled undergarment; and 387 W with use of the long water-cooled undergarment [Kim et al. 2011].
6.3.2 Air-cooled Garments
Air-cooled garments distribute cooling air next to the skin. The total heat exchange from completely sweat-wetted skin when cooling air is supplied to the air-cooled garment is a function of cooling air temperature and cooling airflow rate. Both the total heat exchanges and the cooling power increase with cooling airflow rate and decrease with increasing cooling air inlet temperature. For an air inlet temperature of 10°C (50°F) at 20% RH and a flow rate of 0.28 m3·min-1 (10 ft3·min-1), the total heat exchanges over the body surface would be 233 W in a 29.4°C (84.9°F), 85% RH environment and 180 W in a 51.7°C (125.1°F), 25% RH environment. Increasing the cooling air inlet temperature to 21°C (69.8°F) at 10% RH would reduce the total heat exchanges to 148 W and 211 W, respectively. Either air inlet temperature easily provides 100 W of cooling.
The use of a vortex tube as a source of cooled air for body cooling is applicable in many hot workplace situations. The vortex tube, which is attached to the worker, requires a constant source of compressed air supplied through an air hose. The hose connecting the vortex tube to the compressed air source limits the space within which the worker can operate. However, unless mobility of the worker is required, the vortex tube, even though noisy, may be considered as a simple cooled air source.
6.3.3 Cooling Vests
Currently available cooling vests may contain as many as seventy-two cooling packs made of ice or phase-change materials; cooling packs may also vary in weight and size. These cooling packs are generally secured to the vest by tape, inserted into the vest pockets, or integrated with the vest, which requires freezing the whole vest before use. The cooling provided by each individual cooling pack will vary with time and with its contact pressure with the body surface, plus any heating effect of the clothing and hot environment; thus, the environmental conditions have an effect on both the cooling provided and the duration of time this cooling is provided. In environments of 29.4°C (84.9°F) at 85% RH and 35.0°C (95°F) at 62% RH, a cooling vest can still provide some cooling for up to four hours of operation (about two to three hours of effective cooling is usually the case). However, in an environment of 51.7°C (125.1°F) at 25% RH, any benefit is negligible after about three hours of operation. With 60% of the cooling packs in place in the vest, the cooling provided may be negligible after two hours of operation. Since the cooling vest does not provide continuous and regulated cooling over an indefinite time period, exposure to a hot environment would require redressing with backup cooling packs every two to four hours. Replacing a cooling vest would have to be accomplished when an individual is not in a work situation. However, the cooling is supplied noise-free and independent of any energy source or umbilical cord that would limit a worker’s mobility. The greatest potential for the ice packet vest appears to be for work where other conditions limit the length of exposure, such as short-duration tasks and emergency repairs. The cooling vest is also relatively cheaper than other cooling approaches.
6.3.4. Wetted Overgarments
A wetted overgarment is a wetted cotton terry cloth coverall or a two-piece cotton cover that extends from just above the boots and from the wrists to a V-neck. When used with impermeable protective clothing, it can be a simple and effective auxiliary cooling garment.
Predicted values can be calculated to determine supplementary cooling and the minimal water requirements to maintain the cover wettedness in various combinations of air temperature, RH, and wind speed. Under environmental conditions of low humidity and high temperatures where evaporation of moisture from the wet cover garment is not restricted, this approach to auxiliary cooling can be effective, relatively simple, and inexpensive.
6.3.5 Practical Applications and Limitations
All of these cooling systems have substantial limitations within a work setting. For example, phase-change systems are cheap, but their temperature cannot be controlled and they often do not stay cool long enough to be practical. Ice vests have the same limitation. Indeed, if the system is too cold, this will result in peripheral vasoconstriction, which reduces the heat transfer from the body to the environment. Water cooling systems require that the person be tethered to a system that circulates the cool water, which limits the person’s range of operation. Many of the systems are too heavy or too cumbersome to be practical in a work environment.
However, each system could be used during a rest period where the worker is not actively engaged in work. For example, fire fighters generally take frequent rest breaks because of the exhaustion of the air supply in their SCBA. During these rest breaks, active or passive cooling can be applied to supplement rehydration by removing their ensemble jacket and immersing their arms in cool water. This reduces body core temperature and allows for a more rapid “rehabilitation” during the rest break.
Core body temperature (Tcore) changes relatively slowly, and simply stopping hard work will not result in an immediate decrease in Tcore. Therefore, increasing the rate of heat removal from the body would reduce the chances of heat injury. Using auxiliary cooling systems, such as those described previously, could reduce the time required to lower Tcore, lessen the chances of heat injury, and enable a safer return to work.
6.3.6 Performance Degradation
A variety of options for auxiliary cooling to reduce or eliminate the level of heat stress under indoor and outdoor environmental conditions have been discussed. However, wearing protective clothing systems is also associated with decreases in workers’ performance. Performance decrements are associated with wearing encapsulating protective ensembles, even in the absence of any heat stress [Joy and Goldman 1968]. The majority of decrements result from mechanical barriers to sensory inputs to the wearer and from barriers to communication between individuals. Overall, it is clear that elimination of heat stress allows work to continue, but does not eliminate the constraints imposed by encapsulating protective clothing systems [Joy and Goldman 1968; Nag et al. 1998].
7| Medical Monitoring
Employers should establish a medical monitoring program for workers with occupational exposure to hot environments. The medical monitoring program should include elements of medical screening (secondary prevention) and surveillance (primary prevention) with the goal of early identification of signs or symptoms that may be related to heat-related illness and prevention of adverse outcomes. Early detection of symptoms using medical screening, subsequent treatment, and workplace interventions are intended to minimize the adverse health effects of exposure to hot environments. Medical screening data may also be used for the purposes of medical surveillance to identify work areas, tasks, and processes that require additional prevention efforts.
7.1 Worker Participation
Workers exposed to hot environments who should be included in a medical monitoring program and could receive the greatest benefit include the following:
- Workers exposed to a hot environment above the RAL.
- Workers with personal risk factors, including medical conditions and/or a history of heat-related illness, that put them at a higher risk of heat-related illness.
7.2 Program Oversight
The employer should assign responsibility for the medical monitoring program to a responsible healthcare provider. The responsible healthcare provider should be a qualified physician or other qualified health care professional (as determined by appropriate federal and state laws and regulations) who is informed and knowledgeable about the following:
- Potential workplace exposures to heat and hot environments.
- Administration and management of a medical monitoring program for occupational hazards.
- Identification and management of heat-related illnesses.
- Where respiratory protection is being used, establishment of a respiratory protection program based on an understanding of the requirements of the OSHA respiratory protection standard and types of respiratory protection devices available at the workplace.
7.3 Medical Monitoring Program Elements
Recommended elements of a medical monitoring program for workers at risk for heat-related illnesses and injuries should include worker education, a preplacement medical evaluation, regularly scheduled or periodic follow-up medical evaluations, and reports of incidents of heat-related illnesses and injuries. The purpose of preplacement and periodic medical evaluations of persons working at a particular hot job is (1) to determine if the persons can meet the demands and stresses of the hot job, with reasonable assurance that the safety and health of the individuals and/or fellow workers will not be placed at risk; (2) to inquire whether persons have already suffered from an adverse health effect from heat stress exposure; and (3) to work with management to modify the job as necessary. Based on the findings from these evaluations, more frequent and detailed medical evaluation may be necessary.
7.3.1 Medical Evaluations
7.3.1.1 Preplacement Medical Evaluation
All new workers or workers who are transferring from jobs that do not involve exposure to heat should undergo a preplacement medical evaluation. Unless demonstrated otherwise, it should be assumed that these workers are not acclimatized to work in hot environments. In addition, greater physical demand and exertion in a hot environment can result in more stress on the cardiopulmonary system; therefore screening may need to be more thorough [Ramphal-Naley 2012].
- The responsible healthcare provider should obtain information including:
- A medical and surgical history that in-cludes the cardiac, vascular, respiratory, neurologic, renal, hematologic, GI, and reproductive systems and information about dermatologic, endocrine, musculoskeletal, and metabolic conditions that might affect heat acclimatization or the ability to eliminate heat.
- A complete occupational history, including years of work in each job, the physical and chemical hazards encountered, the physical demands of these jobs, ability to use personal protective equipment, intensity and duration of heat exposure, and nonoccupational exposures to heat and strenuous activities. This history should identify episodes of heat-related disorders and evidence of successful adaptation to work in heat in previous jobs or nonoccupational activities.
- A list of all prescribed and over-the-counter medications used by the worker. The responsible healthcare provider should consider the possible impact of medications that may affect cardiac output, electrolyte balance, renal function, sweating capacity, or autonomic nervous system function. These include diuretics, antihypertensive drugs (e.g., atenolol, betaxolol), sedatives (e.g., barbiturates), antispasmodics, psychotropics, anticholinergics, and drugs that may alter the thirst (haloperidol) or sweating mechanism (e.g., phenothiazines, antihistamines, anticholinergics). See Table 4-2 for additional information on proposed mechanisms of action of drugs implicated in intolerance of heat.
- Information about relevant personal habits, including the use of substances such as alcohol and caffeine which could affect a person’s heat tolerance.
- The direct medical evaluation of the worker should include the following:
- Physical examination. At the discretion of the responsible healthcare provider, candidates who anticipate increased stress of physical activity of the job in a hot environment, those over 50 years of age or those younger than 50 years of age with underlying cardiac risk factors may need to have additional testing (e.g., ECG with interpretation by a cardiologist).
- Clinical chemistry values, determined from urinalysis and any additional clinical chemistry tests deemed appropriate by the responsible healthcare provider.
- Blood pressure evaluation.
- Assessment of the ability of the worker to understand the health and safety hazards of the job, understand the required preventive measures, communicate with fellow workers, and have mobility and orientation capacities to respond properly to emergency situations.
- For workers who must wear respiratory protection or other PPE, a respiratory protection program should be provided. The responsible healthcare provider should assess the worker’s ability to tolerate the total heat stress of a job, which includes the metabolic burdens of wearing and using protective equipment.
- More detailed medical evaluation may be deemed appropriate on a case-by-case basis by the responsible healthcare professional. Communication between the program’s responsible healthcare provider and the worker’s private healthcare provider may be appropriate. The following are examples of findings on preplacement medical evaluation that may indicate the need for further medical evaluation:
- History of ischemic heart disease, valvular heart disease, cardiac arrhythmias, cardiomyopathy, congestive heart failure, congenital heart disease , current use of certain antihypertensive medications indicating the possibility of reduced
- maximum cardiac output, obstructive or restrictive pulmonary disease, diabetes insipidus, diabetes mellitus, renal disease, or rhabdomyolysis.
- The use of prescribed medications that might interfere with heat tolerance or acclimatization (See Table 4-2). An alternate therapeutic regimen may be available that would be less likely to compromise the worker’s ability to work in a hot environment.
- The use of antihypertensive medications that might affect heat tolerance. It may be prudent to monitor blood electrolyte values of workers who follow a salt-restricted diet or who take diuretic medications that affect serum electrolyte levels, especially during the initial phase of acclimatization to heat stress. The use of β-blockers (e.g., atenolol) for the treatment of hypertension may also limit performance on the job.
- A history of skin disease, an injury to a large area of the skin, or an impairment of the sweating mechanism that might impair heat elimination via sweat evaporation from the skin, specific evaluation may be advisable. Some people have defective sweating mechanisms (anhidrosis) and therefore are heat intolerant.
- Obesity may interfere with heat tolerance (see Chapter 4). An obese individual may require special supervision during the acclimatization period. Obesity can be defined as a body mass index (BMI) ≥ 30. Measure height and weight to calculate body mass index according to the following formula:
BMI = weight (in pounds) × 703 / [height (in inches)]2
(Additional information on BMI is available at http://www.cdc.gov/healthyweight/assessing/bmi/adult_bmi/index.html)
7.3.1.2 Periodic Medical Evaluations
All workers in the medical monitoring program should be provided with annual follow-up medical evaluations at regular intervals and at other times as deemed appropriate for the individual worker by the responsible healthcare provider. Evaluations should be based on data gathered in the preplacement medical evaluation, ongoing work history, new or changing symptoms, and when heat exposure change in the workplace. Any worker with signs or symptoms of heat-related illness should be examined immediately and may require more frequent screening and extensive testing. Evaluations should include the following:
- An occupational and medical history update and a physical examination focused on the cardiovascular, respiratory, nervous, and musculoskeletal systems and the skin, performed annually.
- Consideration of specific medical tests when deemed appropriate by the responsible healthcare provider.
7.3.1.3 Written Reports of Medical Findings
Following each medical evaluation, the responsible healthcare provider should give each worker a written report containing the following:
- The results of any medical tests performed on the worker.
- A medical opinion in plain language about any medical condition that would increase the worker’s risk of heat-related illness.
- Recommendations for limiting the worker’s exposure to heat or hot environments.
- Recommendations for further evaluation and treatment of medical conditions detected or for accommodation.
Following each medical evaluation, the responsible healthcare provider should give the employer a written report specifying the following:
- Occupationally pertinent results of the medical evaluation.
- A medical opinion as to whether the worker has any medical conditions that would increase the health risk of exposure to heat in the work environment.
- An estimate of the individual’s tolerance to withstand hot working conditions (see 6.2.3 Enhancing Tolerance to Heat and 6.2.5 Screening for Heat Intolerance).
- An opinion as to whether the worker can perform the work required by the job (i.e., physical fitness for the job).
- Recommendations for reducing the worker’s risk for heat-related illness, which may include use of cooling measures, accommodations or limitations related to work/rest schedules and/or workload, medical recommendations for worksite medical or physiologic monitoring, or reassignment to another job, as warranted.
- A statement that the worker has been informed by the responsible healthcare provider of the results of the medical evaluation and any medical conditions that require further explanation or treatment. The worker is cleared to work in the hot environment so long as no adverse health effects occur.
Specific findings, test results, or diagnoses that have no bearing on the worker’s ability to work in heat or a hot environment should not be included in the report to the employer. Safeguards to protect the confidentiality of the worker’s medical records should be enforced in accordance with all applicable regulations and guidelines.
7.4 Medical Surveillance—Periodic Evaluation of Data
Incidents of heat-related illness should be reported on first aid logs and the OSHA Form 300-A and OSHA Form 301 Incident Report, if required to keep these forms, while following OSHA recordkeeping regulations. For sites under MSHA jurisdiction, MSHA Form 7000-1 may be required. The responsible healthcare provider should evaluate data from incident reporting systems to look for trends in type of injury or illness (e.g., heat-related illness) or job title to target interventions.
To ensure that control practices provide adequate protection to workers in hot areas, data from the medical monitoring program should be evaluated periodically in a systematic manner. Such evaluations may detect repeated incidents on the job, episodes of heat-related disorders, or frequent absences that could be related to heat and could be used as the basis for appropriate worksite interventions. Job-specific clustering of heat-related illnesses or injuries should be followed up by the responsible healthcare provider in collaboration with others responsible for occupational safety and health at the worksite (e.g., industrial hygienists).
7.5 Employer Actions
The employer should ensure that the responsible healthcare provider’s recommended restriction of a worker’s exposure to heat or a hot environment or other workplace hazards is followed and that the RAL is not exceeded without taking additional protective measures. Efforts to encourage worker participation in the medical monitoring program and to promptly report any symptoms to the responsible healthcare provider are important to the program’s success. Medical evaluations performed as part of the medical monitoring program should be provided by the employer at no cost to the participating workers. Where medical removal or job reassignment is indicated, the affected worker should not suffer loss of wages, benefits, or seniority.
7.6 Considerations Regarding Reproduction
7.6.1 Pregnancy
The medical literature provides limited data on potential risks for pregnant women and fertile women with heavy work and/or added heat stress within the permissible limits (e.g., where tre does not exceed 38°C or 100.4°F; see Chapter 5). However, because the human data are limited and because research data from animal experimentation indicate the possibility of heat-related infertility and teratogenicity, a woman who is pregnant or who may potentially become pregnant should be informed by the responsible healthcare provider that absolute assurances of safety during the entire period of pregnancy cannot be provided. The worker should be advised to discuss this matter with her own healthcare provider and discuss necessary accommodations with her supervisor if recommended.
7.6.2 Fertility
Heat exposure has been associated with temporary infertility in both females and males, with the effects being more pronounced in males [Rachootin and Olsen 1983; Levine 1984]. In a study examining the time to pregnancy associated with heat exposure, the time was significantly prolonged in a subgroup of male welders and bakers [Thonneau et al. 1997]. Sperm density, motility, and the percentage of normally shaped sperm can decrease significantly when the temperature of the groin is increased above a normal temperature [Procope 1965; Henderson et al. 1986; Mieusset et al. 1987; Jung and Schuppe 2007]. Available data are insufficient to ensure that the REL protects against such effects. Thus, the responsible healthcare provider should question workers exposed to high heat loads about their reproductive histories.
7.6.3 Teratogenicity
The consequences of hyperthermia during pregnancy depend on the temperature elevation, the duration, and the stage of fetal development at the occurrence [Edwards 2006]. The body of experimental evidence reviewed by Lary [1984] indicates that, in the nine species of warm-blooded animals studied, prenatal exposure of the pregnant females to hyperthermia may result in a high incidence of embryo deaths and in gross structural defects, especially of the head and central nervous system (CNS). An elevation of the body temperature of the pregnant female to 39.5°C to 43°C (103.1°F–109.4°F) during the first week or two of gestation (depending on the animal species) resulted in structural and functional maturation defects, especially of the CNS, although other embryonic developmental defects were also found. It appears that some basic developmental processes may be involved, but selective cell death and inhibition of mitosis at critical developmental periods may be primary factors. Hyperthermia in these experimental studies did not adversely affect the pregnant females, but did adversely affect developing embryos. The length of hyperthermia in the studies varied from 10 minutes a day over a 2- to 3-week period to 24 hours a day for 1 or 2 days.
Retrospective epidemiologic studies have associated hyperthermia lasting from a day or less up to a week or more during the first trimester of pregnancy with birth defects, especially defects in CNS development (e.g., anencephaly) [Lary 1984]. In addition, a hyperthermic episode during pregnancy can result in embryonic death, spontaneous abortion, growth retardation, and other defects of development [Edwards 2006]. However, some of the information on hyperthermia’s effects on a pregnancy stems from women with fevers, so it is difficult to determine whether defects are caused by metabolic changes in the mother due to the infection [Clarren et al. 1979; Pleet et al. 1981; Edwards 2006].
It is important to monitor every hour or so the body temperature of a pregnant worker exposed to total heat loads above the RAL/REL, to ensure that the core body temperature does not exceed 39°C to 39.5°C (102°F–103°F) during the first trimester of pregnancy. Regardless of sex, heat stress exposures with core body temperatures of 39°C (102°F) or greater should be avoided.
8| Basis for the Recommended Standard
The research data and information that served as the basis for the recommended standard are derived from an evaluation of: (a) the available scientific literature; (b) the many new technologies available for assessing heat stress and strain that are currently available; (c) suggested procedures for predicting risk of incurring heat-related disorders, of potentially unsafe acts, and of deterioration of performance; (d) accepted methods for preventing and controlling heat stress; and (e) domestic and international standards and recommendations for establishing permissible heat-exposure limits. This chapter includes a discussion of considerations that provide the basis for or impact the NIOSH recommended criteria for this standard for work in hot environments.
8.1 The NIOSH RALs and RELs
The NIOSH RELs were developed to protect most healthy workers (i.e., those who are physically and medically fit for the level of activity required and wearing the conventional one-layer work clothing ensemble) exposed to environmental and metabolic heat from developing adverse heat-related health effects (i.e., maintain thermal equilibrium). The total heat exposure to unacclimatized workers should be controlled so that unprotected healthy workers are not exposed to combinations of metabolic and environmental heat greater than the applicable RALs; see Figure 8-1. The total heat exposure to acclimatized workers should be controlled so that unprotected healthy workers are not exposed to combinations of metabolic and environmental heat greater than the applicable RELs; see Figure 8-2.
Figure 8-1. Recommended heat stress alert limits (RALs) for unacclimatized workers
Figure 8-2. Recommended heat stress exposure limits (RELs) for acclimatized workers
The RALs and RELs are represented by the following equations, where M represents the metabolic rate in Watts (W):
RAL [°C-WBGT] = 59.9 − 14.1 log10 M [W]
REL [°C-WBGT] = 56.7 − 11.5 log10 M [W]
When working for shorter intervals, workers may be able to work in higher temperatures without adverse heat-related health effects. Therefore, RALs and RELs take work/rest schedules into consideration so are shown for 60, 45, 30, and 15 minute time periods of work; see Figures 8-1 and 8-2. Working for shorter time periods and taking appropriate rest breaks slows down the body’s heat accumulation [Dukes-Dobos and Henschel 1973]. See Tables 6-2 and 6-3 for examples of work/rest schedules.
The NIOSH RALs and RELs were derived using a physiologically based approach originating from a study identifying the work driven zones and environmentally driven zones, and determining the Upper Limit of the Prescriptive Zone (ULPZ) of mine rescue personnel during a series of experiments [Lind 1963; Dukes-Dobos and Henschel 1973; NIOSH 1973, 1986a]. Within the Prescriptive Zone, 95% of individuals are able to thermoregulate given the environmental conditions.
Above the Prescriptive Zone fewer workers are able to thermoregulate and maintain heat balance, leading to rises in the core body temperature. The Effective Temperatures (i.e., the environmental conditions that provide the horizontal axis when plotting the Prescriptive Zone) reported by Lind and others were converted to WBGTs, which then became the vertical axis for the RALs and RELs [Dukes-Dobos and Henschel 1973; NIOSH 1973, 1986a; ACGIH 2009]. The use of WBGT satisfied a set of criteria for developing a heat stress index discussed in Dukes-Dobos and Henschel [1973]: (a) applicability should be proven in practice; (b) all important factors must be included; (c) the measurements and calculations required should be simple and should not require physiological monitoring; (d) the exposure limits should reflect the magnitude of human response; and (e) it should be applicable for setting limits to a wide range of work conditions. The NIOSH WBGT–based limits for workers are similar to those of other national and international standards (see 8.5 Recommendations of U.S. Organizations and Agencies and 8.6 International and Foreign Standards and Recommendations).
The NIOSH RALs and RELs were re-evaluated for this update. No new data were identified to use as the basis for updated RALs and RELs. Studies on unacclimatized and acclimatized individuals demonstrate the validity of the ULPZ at various metabolic rates and WBGTs [Belding and Kamon 1973; Lind 1970; Lind et al. 1970]. It was determined that the current RALs for unacclimatized workers and RELs for acclimatized workers are still protective for most workers. However, as there are many factors that can affect how an individual will respond to hot temperatures, the RALs and RELs may not be protective of everyone (see 3.3 Effects of Clothing on Heat Exchange and 4.1 Physiologic Responses to Heat). For this reason, when assessing the level of heat stress and heat strain in workers, professional judgement is extremely important. ACGIH [2009] summarizes the recent data relevant to occupational exposure limits. Some studies suggest that current OELs may be unnecessarily restrictive [Khogali 1983; Parikh et al. 1976; Rastogi et al. 1992; Tranter 1998]. Ongoing research will provide additional data to continue to evaluate the effectiveness of the RALs and RELs. For summarized information pertaining to the RALs and RELs drawn from all chapters, see 1.1 Workplace Limits and Surveillance.
The NIOSH ceiling limit recommendations for acclimatized and unacclimatized workers were also re-evaluated. Data and scientific evidence were not identified to continue to support the NIOSH ceiling limit recommendations. Acclimatized workers live and work in temperatures above the ceiling limit without adverse health effects. The decision to remove the NIOSH ceiling limit recommendations was supported by scientific peer reviewers of the publicly available draft NIOSH document.
8.2 Estimation of Risks
The ultimate objective of a recommended heat stress standard is to limit the risk of heat-related illness or injury for workers. Risk estimation has become more sophisticated in recent years but still lacks accuracy. Earlier estimation techniques were usually qualitative or, at best, only semiquantitative.
It is generally estimated that 2/1000 workers are at risk for heat stress [Parsons 2003] and that some occupations (firefighting, agriculture, construction, forestry, mining, manufacturing) confer an even greater risk for occupational exposure to heat stress because of the high physical (metabolic) workloads involved, as well as exposure to hot environments and the necessity of wearing PPE [Davies et al. 1976; Slappendel et al. 1993; Kirk and Sullman 2001; Parsons 2003; Maeda et al. 2006; Xiang et al. 2014]. One of the early semiquantitative procedures for estimating the risk of adverse health effects under conditions of heat exposure was designed by Lee and Henschel [1963]. The procedure was based on the known laws of thermodynamics and heat exchange. Although designed for the “standard man” under a standard set of environmental and metabolic conditions, it incorporated correction factors for environmental, metabolic, and worker conditions that differed from standard conditions. A series of graphs was presented that could be used to semiquantitatively predict the percentage of exposed individuals (of different levels of physical fitness and age) likely to experience health or performance consequences under each of 15 different levels of total stress. Part of the difficulty with early attempts to develop procedures for estimating risk was the lack of sufficient reliable industry-experience data to validate the estimates.
Much empirical data have been gathered on the relationship between heat stress and strain (including death from heat stroke) in South Africa’s deep, hot mines. From laboratory data, a series of curves has been prepared to predict the probability of a worker’s body temperature reaching dangerous levels during work under various levels of heat stress [Wyndham and Heyns 1973; Stewart 1979]. Based on these data and epidemiologic data on heat stroke among miners, estimates of probabilities of reaching dangerously high rectal temperatures were made. If a core body temperature of 40°C (104°F) is accepted as the threshold temperature at which a worker is in imminent danger of fatal or irreversible heat stroke, then the estimated probability of reaching this body temperature is 10-6 for a worker exposed to an effective temperature (ET) of 34.6°C (94.3°F), 10-4 at 35.3°C (95.5°F), 10-2 at 35.8°C (96.4°F), and 10-0.5 at 36.6°C (97.9°F). If a body temperature of 38.5 to 39.0°C (101.3–102.2°F) is accepted as the critical temperature, then the ET at which the body temperature reaches these values can also be derived for 10-1 to 10-6 probabilities. These ET correlates were established for conditions with RH near 100%; whether they are equally valid for these same ET values for low humidity has not been determined. Probabilities of body temperature reaching designated levels at various ET values have also been presented for unacclimatized men [Wyndham and Heyns 1973; Strydom 1975; Stewart 1979]. Although these estimates have proven to be useful in preventing heat casualties under the conditions of work and heat found in the South African mines, their application to other workplaces may not be warranted.
A World Health Organization (WHO) scientific group on health factors involved in working under conditions of heat stress concluded that “it is inadvisable for deep body temperature to exceed 38°C (100.4°F) in prolonged daily exposure to heavy work”. The value of 38°C (100.4°F) tre incorporates a modest safety margin, which is required because of the degree of accuracy with which the actual environmental and metabolic heat loads are assessed. In closely controlled conditions, the deep body temperature may be allowed to rise to 39°C (102.2°F)” [WHO 1969]. This does not mean that when a worker’s rectal temperature (tre) reaches 38°C (100.4°F) or even 39°C (102.2°F), the worker will necessarily become a heat casualty. The physiological response to heat stress is quite variable in the human population. In fact, it is well documented that many motivated non-elite distance runners complete marathon-style runs with tre ≥41°C (105.8°F). In addition, tre of 41.9°C (107.4°F) have been measured in soccer players without any physical symptoms or lasting sequelae, whereas there are cases in which heat stroke and death have occurred in individuals with body core temperatures less than 40°C (104°F) who ran less than 10 km under mild environmental conditions [Armstrong et al. 2007b; Taylor et al. 2008]. Thus, heat injury is determined by both core temperature and symptoms, rather than core temperature alone. Although some individuals may not suffer heat illnesses under these conditions, if the tre exceeds 38°C (100.4°F), then the risk of heat casualties in a population increases. Nonthermal contributions to heat injury, such as poor acclimatization, dehydration, alcohol consumption, previous heat injury, age, and drug use, must also be determined [Armstrong et al. 2007b; Taylor et al. 2008].
Some safety margin is also justified by the recent finding that the number of unsafe acts committed by a worker increases with an increase in heat stress [Ramsey et al. 1983]. The data, derived by using safety sampling techniques to measure unsafe behavior during work, showed an increase in unsafe behavioral acts with an increase in environmental temperature. The incidence was lowest at WBGTs of 17°C to 23°C (62.6°F – 73.4°F), whereas a WBGT that exceeds 28°C (82°F) confers the greatest risk of heat stress [Armstrong et al. 2007b]. Unsafe behavior also increased as the level of physical work of the job increased [Ramsey et al. 1983].
8.3 Correlation between Exposure and Effects
The large amount of data published from controlled laboratory studies and from workplace heat stress studies upholds the generality that heat strain increases as heat stress increases. All heat stress/heat strain indices are based on this relationship. This generally holds for heat-acclimatized and heat-unacclimatized individuals, for women and men, for all age groups, and for individuals with different levels of physical performance capacity and heat tolerance. In each case, differences between individuals or between population groups in the extent of heat strain resulting from a given heat stress relate to differences in heat acclimatization and physical work capacity. The individual variability may be large; however, with extreme heat stress, the variability decreases as the limits of the body’s systems for physiologic regulation are reached.
Sophisticated models are available to predict heat strain as a function of heat load and physical activity and are capable of being modified by a variety of confounding factors. These models range from graphic presentations of relationships to programs for handheld and desk calculators and computers [Witten 1980; Kamon and Ryan 1981; Bernard and Pourmoghani 1999]. The strain factors that can be predicted for the average worker are heart rate, body and skin temperature, sweat production and evaporation, skin wettedness, tolerance time, productivity, and required rest allowance. Confounding factors include amount, fit, insulation, and moisture vapor permeability of the clothing worn, physical work capacity, body hydration, and heat acclimatization. From some of these models, it is possible to predict when and under what conditions the physiologic strain factors will reach or exceed values that are considered acceptable from the standpoint of health.
These models are useful in industry to predict when any combination of stress factors is likely to result in unacceptable levels of strain, which then would require introduction of control and correction procedures to reduce the stress. The regression of heat strain on heat stress is applicable to population groups, and, with the use of a 95% confidence interval, it can be applied as a modified form of risk prediction. However, because of the variability in the human physiological response to heat stress (metabolic and/or environmental), the current models do not provide information on the level of heat stress at which one worker in 10, in 1,000, or in 10,000 will incur heat exhaustion, heat cramps, or heat stroke.
8.4 Physiologic Monitoring of Heat Strain
When the NIOSH Criteria for a Recommended Standard: Occupational Exposure to Hot Environments was first published in 1972 (and revised in 1986), physiologic monitoring was not considered a viable adjunct to the WBGT index, engineering controls, and work practices for the assessment and control of workplace heat stress. However, by the time of the 1986 revision, it was proposed that monitoring body temperature and/or the work and recovery heart rate of workers exposed to environmental conditions in excess of the ACGIH TLVs® could be a safe and relatively simple approach [Fuller and Smith 1980, 1981; Siconolfi et al. 1985]. All the heat stress indices assume that most workers will not incur heat-related illnesses or injuries if they are exposed to hot work conditions that do not exceed the permissible value. Inherent in this is the recognition that a small proportion of the exposed workers may develop heat-related illness. The ACGIH TLV® is intended to protect nearly all healthy heat-acclimatized workers at heat stress levels that do not exceed the TLV®.
Physiologic monitoring, such as heart rate and/or core temperature, of heat-exposure workers could help protect all workers, including the heat-intolerant worker exposed at hot worksites. In one field study, the recovery heart rate was taken with the worker seated at the end of a cycle of work from 30 seconds to 1 minute (P1), 1.5 to 2 minutes (P2), and 2.5 to 3 minutes (P3). Oral temperature was measured with a clinical thermometer inserted under the tongue for 4 minutes. The data indicate that, 95% of the time, the oral temperature was below 37.5°C (99.5°F) when the P1 recovery heart rate was 124 bpm or less, and 50% of the time the oral temperature was below 37.5°C (99.5°F) when the P1 was less than 145 bpm. From these relationships, a table was developed for assessing heat strain and suggested remedial actions. If the P3 heart rate is lower than 90 bpm, then the heat stress conditions are satisfactory; if the P3 approximates 90 bpm and/or the P1–P3 recovery is approximately 10 bpm, it indicates that the work level is high but there is little increase in body temperature; if P3 is greater than 90 bpm and/or P1–P3 is less than 10 bpm, it indicates a no-recovery pattern—that is, the heat stress exceeds acceptable levels and corrective actions should be taken to prevent heat injury or illness [Fuller and Smith 1980, 1981]. The corrective actions may be of several types (engineering, work practices, etc.).
In spite of the aforementioned field study findings, recent studies have indicated that body heat is still stored for up to 60 minutes of rest after cessation of work. Although Tre decreases, muscle temperature remains elevated, probably because of sequestration of warm blood in the muscle tissue. Therefore, even in recovery, subjects are still under heat stress [Kenny et al. 2008]. This fact must be taken into consideration when any corrective actions (engineering controls, administrative controls, or the use of PPE) are adopted.
Historically, obtaining recovery heart rates at 1- or 2-hour intervals or at the end of several work cycles during the hottest part of the workday of the summer season presented logistical problems, but available advanced technologies allow many of these problems to be overcome. Wearable sensors, capable of continuous monitoring and recording of physiological responses, have been introduced to the market. Probably the most common example is the heart-rate-recording wristwatch, which is used by many joggers and enables continuous automated heart-rate measurements in real time that are accurate and reliable. The data obtained from the heart rate–recording wristwatches can also be stored, downloaded onto a computer, and analyzed at a later time.
The single-use disposable digital oral thermometer, which is capable of measuring oral temperatures of workers at regular intervals, makes monitoring of body temperature possible under most workplace situations without interfering with the normal work pattern. It would not be necessary to interrupt work to insert the thermometer under the tongue and to remove it after 4 to 5 minutes. However, ingestion of fluids and mouth breathing would have to be controlled for about 15 minutes before an oral temperature is taken. Moreover, oral temperatures are not the most accurate indicator of body core temperature, and such measurements may not be practical for a worker who is nauseated or has already vomited.
A more accurate technology, involving ingestible capsules (CorTemp® Ingestible Core Body Temperature Sensors, Palmetto, FL) capable of recording and telemetering intestinal “core” temperature on a continuous basis, has been in use by the research community for ~20 years and may eventually be used occupationally. The problem with ingestible temperature sensing capsules is that they must be ingested the evening before use and function only until passed from the body during defecation. Another drawback is the cost of the capsules and monitoring equipment.
Other sophisticated wearable physiological sensor systems (e.g., LifeShirt®, VivoMetrics, Ventura, CA) have been or are under development, and one system has been evaluated for its accuracy against standard physiological monitoring systems found in modern laboratories [Coca et al. 2010]. A new system, called the Zephyr BioHarness® (Zephyr Bioharness, British Columbia, Canada), has moved from the research arena to commercial application. This device monitors heart rate, respiratory rate, skin temperature, ECG, body position, vector magnitude, and R-R interval. (The R-R interval is the time between 2 QRS waves in the ECG, in which the R-wave segment of the QRS complex is usually of the greatest magnitude. The time between two R waves corresponds to the heart rate.) These systems and others in development may revolutionize the real-time monitoring of workers in occupations that put them at risk for heat injury.
The advantages of such automated systems are that data could be immediately observed and trends established, from which actions could be initiated to prevent excessive heat strain. Disadvantages are that it takes time to attach and remove the transducers at the start and end of each workday; the transducers for rectal or ear temperature, as well as stick-on electrodes or thermistors, are not acceptable for routine use by some people; and electronic components require careful maintenance for proper operation. Also, the telemetric signals are often disturbed by the electromagnetic fields that may be generated by the manufacturing process. However, devices recently appearing on the market have addressed many of these problems, thus leading the way for common use of wearable physiological monitoring systems in occupations that expose workers to risk for heat injury.
8.5 Recommendations of U.S. Organizations and Agencies
8.5.1 The American Conference of Governmental Industrial Hygienists (ACGIH)
The ACGIH TLV® for heat stress refers to heat stress conditions under which it is believed that nearly all workers may be repeatedly exposed without adverse health effects [ACGIH 2014]. The TLV® goal is to maintain core body temperature within +1°C of normal (37°C), although exceptions can be made under certain circumstances [ACGIH 2014]. ACGIH suggests using a decision-making tree to evaluate the risk of heat stress and strain to workers. The guidance is based on the premises that (1) workers are acclimatized, adequately hydrated, and unmedicated and (2) that a healthy worker can be repeatedly exposed without adverse health effects, and (3) Action Limit guidance is protective of unacclimatized workers.
Those who are more tolerant of working in the heat and are under medical supervision may work under heat stress conditions that exceed the TLV®, but in no instance should the deep body temperature exceed the 38°C (100.4°F) limit for an extended period. However, acclimatized workers may be able to work safely under supervision with a core body temperature not to exceed 38.5°C (101.3°F). The TLV® permissible heat-exposure values incorporate both the environmental heat factors and metabolic heat production. The environmental factors are expressed as the WBGT. ACGIH provides instructions for adjusting the WBGT values on the basis of clothing type. The worker’s metabolic heat production is expressed as work-load category: light work = <180 kcal·h-1; moderate work = 180–300 kcal·h-1; heavy work = 300–415 kcal·h-1; and very heavy work = >520 kcal·h-1.
Along with metabolic heat production, work demands need to be considered, with use of a table that includes WBGT values for 100% work, 75% work/25% rest, 50% work/50% rest, and 25% work/75% rest. If work demands vary or work and rest environments are different, a TWA should be calculated.
ACGIH provides additional guidance for limiting heat strain and for heat stress management. This guidance includes having general and job-specific controls in place and monitoring heart rate, core body temperature, heat stress-related symptoms, profuse sweating rates, and weight loss.
8.5.2 Occupational Safety and Health Administration (OSHA)
Standards Advisory Committee on Heat Stress (SACHS)
In January 1973, the Assistant Secretary of Labor for OSHA appointed a Standards Advisory Committee on Heat Stress (SACHS) to conduct an in-depth review and evaluation of the NIOSH Criteria for a Recommended Standard: Occupational Exposure to Hot Environments and to develop a proposed standard that would establish work practices to minimize the effects of hot environmental conditions on working employees [Ramsey 1975]. The purpose of the standard was to minimize the risk of heat disorders and illnesses of workers exposed to hot environments so that the workers’ well-being and health would not be impaired. The 15 committee members represented worker, employer, state, federal, and professional groups.
The recommendations for a heat stress standard were derived by the SACHS by majority vote on each statement. Any statement that was disapproved by an overwhelming majority of the members was no longer considered for inclusion in the recommendations. The recommendations establish the threshold WBGT values for continuous exposure at three levels of physical work: light, <200 kcal·h-1 (<800 Btu·h-1), 30°C (86°F); moderate, 200–300 kcal·h-1 (804–1200 Btu·h-1), 27.8°C (82°F); and heavy, >300 kcal·h-1 (>1200 Btu·h-1), 26.1°C (79°F), with low air velocities up to 1.52 m·s-1 (300 fpm). These values are similar to the ACGIH TLVs®. When the air velocity exceeds 1.52 m·s-1 (300 fpm), the threshold WBGT values are increased 2.2°C (4°F) for light work and 2.8°C (5°F) for moderate and heavy work. The logic behind this recommendation was that the instruments used for measuring the WBGT index do not satisfactorily account for the increased heat removal when air velocity is increased beyond 1.52 m·s-1 (300 fpm). Kamon and Avellini [1979], presented data that did not support the assumption, because the clothing worn by the worker reduced the cooling effect of increased air velocity. However, under conditions in which heavy protective clothing or clothing with reduced air and/or vapor permeability is worn, higher air velocities may facilitate limited air penetration of the clothing and enhance convective and evaporative heat transfer. A modern WBGT with appropriate anemometry measurements could be used to take air velocity into account for this purpose.
The recommendations of the SACHS contained a list of work practices that were to be initiated whenever the environmental conditions and workload exceed the threshold WBGT values. The threshold WBGT values and work levels are based on a 120-minute TWA. Also included are directions for medical surveillance, training of workers, and workplace monitoring. The threshold WBGT values recommended by the OSHA SACHS were in substantial agreement with the ACGIH TLVs® and the ISO standard. However, the OSHA SACHS recommendations have not been promulgated in an OSHA heat stress standard.
In 2011, OSHA and NIOSH cobranded an infosheet on protecting workers from heat-related illness. This document included risk factors, health problems, first aid, and prevention. In May 2012, OSHA launched a nationwide education and outreach campaign (Water. Rest. Shade.) to educate workers and employers about the hazards of heat and preventing heat-related illnesses. OSHA worked with California OSHA (Cal/OSHA) and adapted many of that state’s campaign materials for national purposes. Many of these materials target at-risk populations and those with limited English proficiency. OSHA has also partnered with the National Oceanic and Atmospheric Administration (NOAA) on weather service alerts, requesting that worker safety precautions be included when extreme heat alerts are issued [OSHA-NIOSH 2011; OSHA 2012b]. A smart phone downloadable application was developed by OSHA to provide a way for employers or workers to calculate the heat index and view risk levels as well as protective measures [OSHA 2012a]. In 2014, the ongoing OSHA heat campaign also began to emphasize the importance of including acclimatization in heat illness prevention programs. OSHA has also been making efforts to reach out to state and local partners, national and local conferences, consultation programs, employers, trade associations, unions, community and faith-based organizations, consulates, universities, and health care and safety professionals.
8.5.2.1 Cal/OSHA
In 2005, the California Standards Board enacted emergency heat regulations based on a Cal/OSHA investigation. Cal/OSHA drafted the Heat Illness Prevention standard in collaboration with the Labor and Workforce Development Agency, worker and employer communities, the Standards Board, and other interested parties; and in 2006, the state of California adopted the heat stress standard [Wilson 2008]. The standard (Title 8, Chapter 4, § 3395, Heat Illness Prevention) applies to all outdoor places of employment and addresses access to potable drinking water; access to shade; high heat procedures; and employee and supervisor training. Concerns over the Cal/OSHA standard have included a lack of heat stress threshold that accounts for humidity and lack of mandatory rest breaks.
In 2010, Cal/OSHA used a heat-related illness prevention campaign to target low-wage, non–English speaking outdoor workers to reduce heat-related illnesses and fatalities. The campaign included media ads, radio spots, promotional items, posters, DVDs, postcards, training kits, and community and employer outreach and training. A subsequent evaluation of the campaign concluded that a sustained effort is needed in order to achieve long-term behavior change. Enforcement, as well as education, is important to producing an enduring impact and changing longstanding attitudes and cultural norms [Cal/OSHA 2010]. In addition, the report concluded that many immigrant workers are afraid of contacting government agencies about hazards at work, so Cal/OSHA is planning a hotline that will employ community sources to handle phone calls. Cal/OSHA launched another campaign in 2012 to prevent worker deaths and illnesses due to heat exposure in all outdoor workplaces in California.
8.5.3 American Industrial Hygiene Association (AIHA)
AIHA states that the best way to protect workers from the stresses of thermal environments is to help workers and supervisors understand the fundamentals of thermoregulation and exposure control [AIHA 2003]. AIHA’s The Occupational Environment: Its Evaluation, Control, and Management [AIHA 2003] contains a thorough overview of many of the heat exposure limits available, including WBGT recommendations, time-weighted averages, NIOSH recommendations, ACGIH TLVs®, and ISO recommendations. AIHA notes that the metabolic heat assumptions and WBGT threshold limit proposals are very similar:
- resting, 32°C–33°C;
- light, 30°C;
- moderate, 27°C–28°C;
- heavy, 25°C–26°C; and
- very heavy, 23°C–25°C.
See Table 5-1. AIHA concludes that the WBGT threshold values are basically equivalent.
8.5.4 The Armed Services
The 2003 publication entitled Heat Stress Con-trol and Heat Casualty Management (TBMED 507/AFPAM 48-52 (I)) addresses in detail the procedures for assessment, measurement, evaluation, and control of heat stress and the recognition, prevention, and treatment of heat-related illnesses and injuries [DOD 2003]. The document may be applicable to many industrial- and outdoor worker–type settings. The WBGT index is used for measurement and assessment of the environmental heat load.
The Navy Environmental Health Center [2007] developed a technical manual (NEHC-TM-OEM 6260.6A) entitled Prevention and Treatment of Heat and Cold Stress Injuries. This document includes information on risk factors, hydration status, heat stress injuries, treatment, and follow-up. Like the aforementioned 2003 publication, this document uses the WBGT index.
In addition, both TBMED 507/AFPAM 48-52 and NEHC-TM-OEM 6260.6A include examples of water intake tables based on WBGT, level of work, and number of minutes worked or the work/rest schedule (see Table 8-1).
8.5.5 American College of Sports Medicine (ACSM)
In 2007, the American College of Sports Medicine (ACSM) published a revised position statement, Exertional Heat Illness During Training and Competition [Armstrong et al. 2007b]. To be competitive, the long distance runner must be in excellent physical condition, exceeding the physical fitness of most workers. For long distance races, such as marathons, the fastest competitors run at 12 to 15 miles per hour, which is classified as extremely hard physical work. When the thermal environment reaches even moderate levels, overheating can be a problem.
To reduce the risk of heat-related injuries and illnesses, the ACSM has prepared a list of recommendations to serve as advisory guidelines to be followed during distance running when the environmental heat load exceeds specific values. These recommendations include the following: (1) races of 10 km or longer should not be conducted when the WBGT exceeds 28°C (82.4°F); (2) all summer events should be scheduled for early morning, ideally before 8 a.m. or after 6 p.m.; (3) race sponsors must provide fluids; (4) runners should be encouraged to drink 300–360 mL of fluids 10 to 15 minutes before the race; (5) fluid ingestion at frequent intervals during the race should be permitted, with water stations at 2- to 3-km intervals for races 10 km or longer, and runners should be encouraged to drink 100–200 mL at each water station; (6) runners should be instructed on recognition of early signs and symptoms of heat-related illness; and (7) provisions should be made for the care of heat-related illness cases.
In these recommendations, the WBGT is the heat stress index of choice. When the “red flag” high-risk WBGT index value reaches 23°C to 28°C (73.4°F–82.4°F) all runners must be made aware that heat injury is possible, and any person particularly sensitive to heat or humidity should be advised to not run. An “amber flag” indicates moderate risk with a WBGT of 18°C to 23°C (64.4°F–73.4°F). It is assumed that the air temperature, humidity, and solar radiation are likely to increase during the day.
8.5.6 Washington State Department of Labor and Industries
In 2008, Washington State Department of Labor and Industries enacted the Outdoor Heat Exposure Rule, WAC 296-62-095. The rule applies to all employers with employees performing work in an outdoor environment from May 1 through September 30 annually. The rule also stipulates this is applicable only if employees are exposed to temperatures at or above 31.7°C (89°F), wearing double-layer woven clothes (e.g., coveralls, jackets, and sweatshirts) in temperatures at or above 25°C (77°F), or wearing nonbreathing clothes (e.g., vapor barrier clothing or PPE such as chemical resistant suits) in temperatures at or above 11.1°C (52°F) [Washington State Legislature]. These temperature limits were based on prevalent dew point temperatures across Washington, which are lower than many other U.S. locations, so these limits might not be generalizable to other areas of the United States.
The Outdoor Heat Exposure Rule states that an outdoor heat exposure safety program must be addressed in the employer’s written accident prevention program. Employers must also encourage their workers to drink water or other acceptable beverages and must provide at least 1 quart of water per hour for each employee. Employers must also relieve from duty any workers showing signs or symptoms of heat-related illness and provide a sufficient means to reduce their body temperature. The rule also states the necessity of providing appropriate training to workers before they begin work in excessive heat, as well as the need for appropriate training of supervisors.
8.5.7 Mine Safety and Health Administration (MSHA)
In 1976, MSHA published an Information Report, Heat Stress in Hot U.S. Mines and Criteria for Standards for Mining in Hot Environments [MSHA 1976]. MSHA also published a revised Safety Manual Number 6, Heat Stress in Mining, in 2001 [MSHA 2001]. MSHA notes a hot worksite can be defined as a WBGT exceeding 79°F (26°C). Heat problems can be reduced in mines by using administrative and engineering controls, advisable work practices, appropriate clothing, and PPE. The following work practices are recommended:
- Increasing workers’ heat tolerance by heat acclimatization, and by increasing their physical fitness.
- A work/rest schedule with frequent breaks and reasonably short work periods.
- Pacing a task.
- Performing heavy tasks in cooler areas or at cooler times.
- Rotating personnel on hot jobs, providing readily accessible cooler rest areas, cool drinking water (50°F–60°F [10°C–15°C]), and encouraging all workers to drink a cup of water every 15 to 20 minutes.
- For persons not on a restricted salt diet by physician’s orders, a heavier use of salt at meals and drinking slightly salted water (about 1 tablespoon salt to 15 quarts of water).
MSHA outlines two different acclimatization schedules based on whether the miner is new or has acclimatized and been on leave for 9 or more consecutive days (see Table 8-2).
Mine supervisors and workers should know the signs and symptoms of heat-related illness, how to administer first aid, and how to reduce heat stress. In addition, new miners should be warned against trying to keep up with their acclimatized co-workers. In a medical examination for all workers assigned to a hot job for the first time, the physician should examine the heart, blood vessels, kidneys, liver, glands of internal secretion, respiratory system, and skin. Proper mine planning, ventilation, and air conditioning may reduce heat stress; and canopies can reduce solar heat and shielding can control radiant heat. Covered ditches and insulated piping can move hot ground water to the surface. Efficient blasting procedures will reduce additional heat into the air.
8.6 International and Foreign Standards and Recommendations
International organizations and national organizations outside of the United States have developed and published standards, recommendations, and guidelines for limiting the exposure of workers to potentially harmful levels of occupational heat stress. These documents range from official international and national position standards to unofficial suggested practices and procedures and to unofficially sanctioned guidelines proposed by institutions, research groups, or individuals concerned with the health and safety of workers under conditions of high heat load. Most of these guidance documents have in common the use of (1) the WBGT as the index for expressing the environmental heat load and (2) some method for estimating and expressing the metabolic heat production. The permissible total heat load is then expressed as a WBGT value for all levels of physical work, ranging from resting to very heavy work.
8.6.1 The International Organization for Standardization (ISO)
As of 2012, the International Organization for Standardization (ISO) has members from 164 countries that develop standards using a consensus-based approach. ISO standards are developed through a multi-stakeholder process with technical committees created from industry experts, consumer associations, academia, non-government organizations, and governments [ISO 2012].
8.6.1.1 ISO 7243
In 1989, the ISO revised ISO 7243: Hot Environments—Estimation of Heat Stress on Working Man, Based on the WBGT-Index (Wet Bulb Globe Temperature) [ISO 1989]. ISO 7243 can be used to assess a hot environment with a simple method based on the WBGT. It can easily be used in a workplace environment for evaluating the stresses on an individual [ISO 1989].
The ISO standard index values are based on the assumption, as are most other recommended heat stress limit values, that the worker is a normal, healthy individual, physically fit for the level of activity being done, and wearing standard summer-weight work clothing with a thermal insulation value of about 0.6 clo (not including the still-air-layer insulation).
The environmental measurements specified in the ISO standard for calculation of the WBGT are (1) air temperature, (2) natural wet bulb temperature, and (3) black globe temperature. From these, WBGT index values can be calculated or can be obtained as a direct integrated reading with some types of environmental measuring instruments. The measurements must, of course, be made at the place and time of the worker’s exposure.
8.6.1.2 ISO 7933
ISO 7933, entitled Ergonomics of the Thermal Environment: Analytical Determination and Interpretation of Heat Stress Using Calculation of the Predicted Heat Strain, describes a method for predicting the sweat rate and the internal core temperature that the human body will develop in response to the working conditions [ISO 2004b]. The main objectives of ISO 7933:2004 include (1) the evaluation of the thermal stress in conditions likely to lead to excessive core temperature increase or water loss for the standard subject and (2) the determination of exposure times with which the physiological strain is acceptable (no physical damage is to be expected).
8.6.1.3 ISO 8996
ISO 8996, entitled Ergonomics of the Thermal Environment: Determination of Metabolic Heat, last revised in 2004, specifies methods for determining the metabolic rate in a working environment, assessing working practices, and determining the energetic cost of a job or activity [ISO 2004c].
8.6.1.4 ISO 9886
ISO 9886, entitled Ergonomics: Evaluation of Thermal Strain by Physiological Measurements, describes methods for measuring the physiological strain on humans by considering four parameters [ISO 2004a]. ISO 9886 provides the principles and practical guidance for measuring body core temperature, skin temperatures, heart rate, and body mass loss.
8.6.1.5 ISO 9920
ISO 9920, entitled Ergonomics of the Thermal Environment: Estimation of Thermal Insulation and Water Vapour Resistance of a Clothing Ensemble, specifies methods for estimating the thermal characteristics (resistance to dry heat loss and evaporative heat loss) for a clothing ensemble on the basis of values for known garments, ensembles, and textiles [ISO 2007].
8.6.2 Canada
The Canadian Centre for Occupational Health and Safety uses two types of exposure limits: occupational exposure limits to protect industrial workers and thermal comfort limits to protect office workers. Some Canadian jurisdictions have adopted ACGIH TLVs® as occupational exposure limits and others use them as guidelines to control heat stress in the workplace. Thermal comfort limits are set by the CSA Standard CAN/CSA Z412-00 (R2005), Office Ergonomics, which gives acceptable ranges of temperature and RH for offices [Canadian Centre for Occupational Health and Safety 2011]. In addition to the standards, Health Canada, concerned with the changing climate and longer, more intense heat events, has been developing extreme heat event–related materials to educate and raise awareness among workers and the general public.
8.6.3 Japan
In Japan, the Society for Occupational Health decides the heat and cold stress threshold limit values, and the thermal standard for offices is decided by the Ministry of Health, Labor and Welfare [Tanaka 2007]. These standards are based on acclimatized, healthy male workers who wore normal working clothes for summer and drank adequate salt water (salt concentration of around 0.1%). The working period was either continuous for 1 hour or intermittent for 2 hours (See Table 8-3).
9| Indices for Assessing Heat Stress and Strain
During the past 75 years, several indices have been developed for assessing and/or predicting the level of heat stress and/or strain that a worker might experience when working at hot jobs. Some are based on the measurements of a single environmental factor (wet bulb), whereas others incorporate all of the important environmental factors (dry bulb, wet bulb, and mean radiant temperatures and air velocity). For all of the indices, either the level of metabolic heat production is directly incorporated into the index or the acceptable level of index values varies as a function of metabolic heat production.
To have workplace application, an index must meet the following minimum criteria:
- Feasibility and accuracy must be proven with use.
- All important factors (environmental, metabolic, clothing, physical condition, etc.) must be considered.
- Required measurements and calculations must be simple.
- The measuring instruments and techniques applied should result in data that accurately reflect the worker’s exposure but do not interfere with the worker’s performance.
- Index exposure limits must be supported by corresponding physiologic and/or psychological responses that reflect an increased risk to safety and health.
- The index must be applicable for setting limits under a wide range of environmental and metabolic conditions.
The measurements required, advantages, disadvantages, and applicability to routine workplace use of some of the more frequently used heat stress/heat strain indices will be discussed under the following categories: (1) Direct Indices, (2) Rational Indices, (3) Empirical Indices, and (4) Physiological Monitoring.
9.1 Direct Indices
9.1.1 Dry Bulb Temperature
The dry bulb temperature (ta) is commonly used for estimating comfort conditions for sedentary people wearing conventional indoor clothing (1.4 clo, including the surface air layer). With light air movement and RH of 20% to 60%, air temperatures of 22°C to 25.5°C (71.6°F–77.9°F) are considered comfortable by most people. If work intensity is increased to moderate or heavy work, the comfort air temperature is decreased about 1.7°C (3°F) for each 25 kcal (100 Btu or 29 W) increase in the hourly metabolic heat production. Conversely, if the air temperature and/or the metabolic heat production is progressively increased above the comfort zone, then the level of heat stress and heat strain will increase.
Dry bulb temperature is easily measured, but its use when the temperature is above the comfort zone is not justified, except for work situations where the worker is wearing completely vapor- and air-impermeable encapsulating protective clothing. Even under these conditions, appropriate adjustments must be made when significant solar and long wave radiation are present [Goldman 1981].
9.1.2 Wet Bulb Temperature
The psychrometric wet bulb temperature (tpwb) may be an appropriate index for assessing heat stress and predicting heat strain under conditions where radiant temperature and air velocity are not large factors and where tpwb approximates ta (high humidities). For normally clothed individuals at low air velocities, a wet bulb temperature of about 30°C (86°F) is the upper limit for unimpaired performance on sedentary tasks and 28°C (82.4°F is the upper limit) for moderate levels of physical work. As tpwb increases above these threshold values, performance deteriorates and accidents increase. The wet bulb temperatures under these hot, humid conditions have been used to predict risk of heat stroke occurring in South African and German mines [Stewart 1979].
Wet bulb temperature is easy to measure in industry with a sling or aspirated psychrometer, and it should be applicable in any hot, humid situation where tpwb approaches skin temperature, radiant heat load is minimal, and air velocity is light.
9.2 Rational Indices
9.2.1 Operative Temperature
The operative temperature (to) expresses the heat exchange between a worker and the environment by radiation and convection in a uniform environment as it would occur in an actual workplace environment. The to can be derived from the heat-balance equation where the combined convection and radiation coefficient is defined as the weighted sum of the radiation and convection heat-transfer coefficients, and it can be used directly to calculate heat exchange by radiation and convection. The to considers the intrinsic thermal efficiency of the clothing. Skin temperature must be measured or assumed. The to presents several difficulties. For convective heat exchange, a measure of air velocity is necessary. Not included are the important factors of humidity and metabolic heat production. These omissions make its applicability to routine workplace use somewhat limited.
9.2.2 Belding-Hatch Heat Stress Index
The Belding and Hatch Heat Stress Index (HSI) [Belding and Hatch 1955] has had wide use in laboratory and field studies of heat stress. One of its most useful features is the table of physiological and psychological consequences of an 8-hour exposure at a range of HSI values. The HSI is essentially a derivation of the heat- balance equation that includes the environmental and metabolic factors. It is the ratio (times 100) of the amount of body heat that is required to be lost to the environment by evaporation for thermal equilibrium (Ereq), divided by the maximum amount of sweat evaporation allowed through the clothing system that can be accepted by the environment (Emax). It assumes that a sweat rate of about one liter per hour over an 8-hour day can be achieved by the average, healthy worker without harmful effects. This assumption, however, lacks epidemiologic proof. In fact, data indicate that a permissible eight liters per 8-hour day of sweat production is too high and, as the 8-hour sweat production exceeds five liters, workers will dehydrate more than 1.5% of the body weight, thereby increasing the risk of heat-related illness and injuries. The graphic solution of the HSI that has been developed assumes a 35°C (95°F) skin temperature and a conventional long-sleeved shirt and trouser ensemble. The worker is assumed to be in good health and acclimatized to the average level of daily heat exposure.
The HSI is not applicable for very high heat stress conditions. It also does not correctly differentiate between the heat stress resulting from hot dry or hot humid conditions. The heat stress resulting from metabolic vs. environmental heat is not differentiated. Because Ereq/Emax is a ratio, the absolute values of the two factors are not addressed, that is, the ratio for an Ereg and Emax of 300 or 500 or 1,000 each would be the same (100); yet the heat stress would be expected to be greater at the higher Ereq and Emax values. Therefore, if one uses a ratio of the evaporation required for thermoregulation to the maximal evaporation that can be achieved by the person, the ratio does not take into consideration the actual increase in heat stress imposed with a greater requirement for evaporation required for thermoregulation.
The environmental measurements require data on air velocity which provide, at best, an approximation under industrial work situations; in addition, ta, twb, and tr must be measured. Metabolic heat production must also be measured or estimated. The measurements are, therefore, difficult and/or time-consuming, which limits the application of the HSI as a field monitoring technique.
The heat transfer coefficients used in the original HSI have been revised by McKarns and Brief as a result of observations on clothed subjects [McKarns and Brief 1966]. Their modification of the HSI nomograph facilitates the practical use of the index, particularly for the analysis of factors contributing to the heat stress. The McKarns and Brief modification also permits the calculation of allowable exposure time and rest allowances at different combinations of environmental and metabolic heat loads; however, the accuracy of these calculations is affected by the limitations of the index mentioned above. HSI programs for programmable handheld calculators are available.
9.2.3 Skin Wettedness (%SWA)
Several of the rational heat stress indices are based on the concept that, in addition to the sweat production required for temperature equilibrium (Ereq) and the maximum amount of sweat that can be evaporated (Emax), the efficiency of sweat evaporation will also affect heat stress. The less efficient the evaporation, the greater will be the body surface area that has to be wetted with sweat to maintain the required evaporative heat transfer; the ratio of wetted to nonwetted skin area times 100% (SWA = Ereq/Emax).
This concept of wettedness gives new meaning to the Ereq/Emax ratio as an indicator of heat strain under conditions of high humidity and low air movement where evaporation is restricted [Goldman 1973, 1978; Gonzalez et al. 1978; Candas et al. 1979; Kamon and Avellini 1979; ISO 1982b]. The skin wettedness indices consider the variables basic to heat balance (air temperature, humidity, air movement, radiative heat, metabolic heat, and clothing characteristics) and require that these variables be measured or calculated for each workplace situation where an index will be applied. These measurements require exacting and time-consuming procedures. In addition, wind speed at the worksite is difficult to measure with any degree of reliability; at best, it can generally be only an approximation. These indices are satisfactory as a basis for calculating the magnitude of heat stress and strain and for recommending engineering and work practice controls; however, as procedures for routine environmental monitoring, they are too complicated, require considerable recording equipment, and are time-consuming.
9.3 Empirical Indices
Some of the earlier and most widely used heat stress indices are those based upon objective and subjective strain response data obtained from individuals and groups of individuals exposed to various levels and combinations of environmental and metabolic heat stress factors.
9.3.1 The Effective Temperature (ET, CET, ET*, and P4SR)
The effective temperature (ET) index is the first and, until recently, the most widely used of the heat stress indices. The ET combines dry bulb and wet bulb temperatures and air velocity. In a later version of the ET, the Corrected Effective Temperature (CET), the black globe temperature (tg) is used instead of ta to take the effect of radiant heating into account. The index values for both the ET and the CET were derived from subjective impressions of equivalent heat loads between a reference chamber at 100% humidity and low air motion and an exposure chamber where the temperature and air motion were higher and the humidity lower. Recently a newer version of the effective temperature (ET*) was developed that uses 50% reference RH rather than 100% reference RH used for the ET and CET. The ET* has all the limitations of the rational heat stress indices mentioned previously; however, it is useful for calculating ventilation or air-conditioning requirements for maintaining acceptable conditions in buildings.
The ET and CET have been used in studies of physical, psychomotor, and mental performance changes as a result of heat stress. In general, performance and productivity decrease as the ET or CET exceeds about 30°C (86°F). The WHO has recommended that for heat-unacclimatized individuals ET or CET values that exceed 30°C (86°F) for sedentary activities, 28°C (82.4°F) for moderate work, and 25.5°C (79.7°F) for hard work are unacceptable. For fully heat-acclimatized individuals, the recommended limits are increased about 2°C (3.5°F). The data on which the original ET was based came from studies on sedentary subjects exposed to several combinations of ta, tpwb, and Va, all of which approximated or slightly exceeded comfort conditions. The responses measured were subjective impressions of comfort or equal sensations of heat, which may or may not be directly related to values of physiologic or psychologic strain. In addition, the sensations were the responses to transient changes. The extrapolation of the data to various amounts of metabolic heat production has been based on workplace experience. The ET and CET have been criticized because they seem to overestimate the effects of high humidity and underestimate the effects of air motion and thus tend to overestimate the heat stress.
In the hot, humid mines of South Africa, heat-acclimatized workers doing hard physical work showed a decrease in productivity beginning at ET of 27.7°C (81.9°F) (at 100% RH with minimal air motion), which is approximately the reported threshold for the onset of fatal heat stroke during hard work [Wyndham 1974; Strydom 1975]. These observations lend credence to the usefulness of the ET or CET as a heat stress index in mines and other places where the humidity is high and the radiant heat load is low.
The limitations of ET have led to the development of the concept of the four-hour sweat rate (P4SR). The P4SR was developed in environmental chambers at the National Hospital for Nervous Diseases in London and formally evaluated in Singapore for seven years [Macpherson 1960; Parsons 2003]. The P4SR is the approximate amount of sweat secreted by presumed healthy young men acclimatized to a particular environment for a duration of 4 hours. This value is used as an index value of sweating but not as a predictor of the specific amount of sweat produced by a group of subjects. The P4SR is, therefore, an empirical index or measure that can be obtained by the following steps:
- Collect twb, tg, and ta.
- If tg ≠ ta, then increase the wet bulb temperature by 0.4 (tg – ta) °C.
- If the metabolic rate M is >63 Wm-2, then increase the wet bulb temperature by the amount indicated in a special nomogram.
- If the subjects are clothed, increase the wet bulb temperature by 1.5 Iclo (°C).
From these empirical measures, the basic four-hour sweat rate (B4SR) can be determined from a nomogram developed from this analysis. From this, the nomogram is used to calculate P4SR. (Nomogram can be found in Parsons [2003], Figure 10.4.)
Since it was determined that the sweat rate outside the prescriptive zone was not an adequate indicator of heat strain, the P4SR has been used to make adjustments to account for this inadequate level of prediction of heat strain. Although the P4SR is useful for defined conditions, its applicability is limited in many workplace settings since the effects of clothing on heat stress are oversimplified. Therefore, the P4SR is most useful as a heat storage index [Parsons 2003].
9.3.2 The Wet Bulb Globe Temperature (WBGT)
The WBGT index was developed in 1957 as a basis for environmental heat stress monitoring to control heat casualties at military training camps. Its advantages are that the measurements are few and easy to make; the instrumentation is simple, relatively inexpensive, and rugged; and the calculations of the index are straightforward. The data obtained from the WBGT can be collected as a continuous recording by a Squirrel data logging system (Grant Instruments, Ltd., Cambridgeshire, UK) [Åstrand et al. 2003]. For indoor use, only two measurements are needed: natural wet bulb and black globe temperatures (dry heat). For outdoors in sunshine, the air temperature must also be measured.
The calculation of the WBGT for indoors is
WBGT = 0.7tnwb + 0.3tg
and the calculation of the WBGT for out- doors is
WBGT = 0.7tnwb + 0.2tg + 0.1ta.
The WBGT combines the effect of humidity and air movement (in tnwb), air temperature and radiation (in tg), and air temperature (ta) as a factor in outdoor situations in the presence of sunshine. If there is no radiant heat load (no sunshine), then the tg reflects the effects of air velocity and air temperature. Commercially available WBGT measuring instruments give ta, tnwb, and tg separately or as an integrated WBGT in a form for digital readouts. A printer can be attached to provide tape printouts at selected time intervals for WBGT, ta, tnwb, Va, and tg values.
The application of the WBGT index for determining training schedules for military recruits during the summer season resulted in a striking reduction in heat casualties [Minard 1961]. This dramatic control of heat casualty incidence stimulated its application to hot workplace situations.
In 1972, the first NIOSH Criteria for a Recommended Standard: Occupational Ex- posure to Hot Environments [NIOSH 1972] recommended the use of the WBGT index for monitoring workplace heat stress. The rationale for choosing the WBGT and the basis for the recommended guideline values was described in 1973 [Dukes-Dobos and Henschel 1973]. The WBGT was used as the index for expressing environmental heat load in the ACGIH TLVs® for heat stress, adopted in 1974 [ACGIH 1985]. Since then, the WBGT has become the index most frequently used and recommended for use throughout the world, including its use in the ISO document Hot Environments: Estimation of Heat Stress on Working Man Based on the WBGT Index (Wet Bulb Globe Temperature) 1982 [ISO 1982a] (see Chapter 8, Basis for the Recommended Standard, for further discussion of the adoption of the WBGT as the recommended heat stress index). However, when impermeable clothing is worn, the WBGT is not a relevant index because evaporative cooling (wet bulb temperature) will be limited. The air temperature or adjusted dry bulb temperature is the pertinent factor in the determination of heat transfer from the human body to the external environment.
The WBGT index meets the criteria of a heat stress index that are listed earlier in this chapter. In addition to the WBGT-based occupational exposure limit recommendations for continuous work in a hot environment, recommendations have also been made for limiting WBGT heat stress when 25%, 50%, and 75% of each working hour is at rest (ACGIH-TLVs®, OSHA, AIHA). Regulating work time in the heat (allowable exposure time) is a viable alternative technique for permitting necessary work to continue under heat stress conditions that would be intolerable for continuous exposure.
9.3.3 Wet Globe Temperature (WGT)
Next to the ta and twb, the wet globe thermometer, also known as the Botsball, is the simplest, most easily read, and most portable of the environmental measuring devices. The wet globe thermometer consists of a thermometer inside a hollow 3-inch copper sphere covered by two layers of black cloth, which is kept at 100% wettedness from a water reservoir. The sensing element of a thermometer is located at the inside center of the copper sphere, and the temperature inside the sphere is read on a dial on the end of the stem.
Presumably, the wet sphere exchanges heat with the environment by the same mechanisms that a nude person with totally wetted skin would in the same environment; that is, heat exchange by convection, radiation, and evaporation are integrated into a single instrument reading [Botsford 1971]. The stabilization time of the instrument ranges from about 5 to 15 minutes, depending on the magnitude of the heat-load differential (5 minutes for 5°C [9°F] and 15 minutes for >15°C [59°F]). However, a study that investigated the relationship between the Botsball and WBGT in varied environmental conditions indicated that if good estimates of wind speed, humidity and radiant heat are available, WGT can predict WBGT with +/-0.4 degrees C with a 90% confidence level but this does not hold true when there is limited knowledge of the of environment conditions (e.g., humidity, heat, wind speed) [Ciricello and Snook 1977].
The WGT has been compared to the WBGT in many laboratory studies and field situations [Ciricello and Snook 1977; Johnson and Kirk 1980; Beshir 1981; Beshir et al. 1982; Parker and Pierce 1984]. In general, the correlation between the two is high (r = 0.91 - 0.98); however, the relationship between the two is not constant for all combinations of environmental factors. Correction factors ranging between 1°C (1.8°F) and 7°C (12.6°F) have been suggested. A simple approximation of the relationship is WBGT = WGT + 2°C for conditions of moderate radiant heat and humidity (22°C, 50% relative humidity). These approximations are probably adequate for general monitoring in industry. If the WGT shows high values, it should be followed with WBGT or other detailed measurements. The WGT, although adequate for screening and monitoring, does not yield data for solving the equations for heat exchange between the worker and the workplace environment, but a color-coded WGT display dial provides a simple and rapid indicator of the level of heat stress.
9.3.4 Universal Thermal Climate Index (UTCI)
A more recent model has been developed to determine the thermal stress imposed by environmental conditions on the human body [Blażejczyk et al. 2013]. In this model, the human body is divided into two interacting thermoregulatory systems which respond to the environment (an active controlling and a passive controlled system). This model represents the human body as a complex series of segments containing information on anatomical and physiological properties which include heat balance. The model predicts the thermoregulatory responses (e.g., cutaneous blood flow, shivering thermogenesis, sweating responses) involved in human heat balance under various environmental conditions. The model defines UTCI as the air temperature of the reference condition causing the same model responses as the actual conditions. The deviation or offset from UTCI depends on actual values of air temperature and mean radiant temperature, wind velocity and relative humidity. Thus, the model takes into consideration not just the environmental conditions but the physiological responses to those conditions. Additional information and a UTCI calculator are available at http://www.utci.org.
9.3.5 Psychrometric Chart
In the event that the methods for obtaining estimates of environmental heat stress as described in this section are unavailable, good estimates of environmental heat stress can still be determined. A psychrometric chart can be used to determine the environmental heat stress (see 5.1.5 Psychrometric Chart). The psychrometric chart is a graphical representation of the relationships among dry bulb temperature, wet bulb temperature, RH, vapor pressure, and dew point temperature. If any two of these variables are known, any of the others can be determined from the psychrometric chart.
9.4 Physiologic Monitoring
The objectives of a heat stress index are twofold: (1) to provide an indication of whether a specific total heat stress will result in an unacceptably high risk of heat-related illness or injuries and (2) to provide a basis for recommending control procedures. The physiologic responses to an increasing heat load include increases in heart rate, core body temperature, skin temperature, and sweat production. In a specific situation, any one or all of these responses to heat load may be elicited. The magnitude of the response(s) generally reflects the total heat load. An advantage of physiological monitoring is that it measures the individual’s responses to the environmental and metabolic heat load rather than extrapolating from the environmental conditions and the estimated work load. The individual integrates the stress of the heat load from all sources, and the physiologic responses (strain) to the heat load are the biological corrective actions designed to counteract the stress and thus permit the body to maintain an optimal internal temperature. Acceptable limits to increases in physiologic responses to heat stress have been recommended by several investigators [WHO 1969; Fuller and Smith 1980, 1981; Bernard and Kenney 1994; Logan and Bernard 1999; Malchaire et al. 2000]. Measuring one or more of the physiologic responses (heart rate and/or core temperature) during work has been recommended and, in some industries, is used to ensure that the heat stress to which the worker is exposed does not result in unacceptable strain [Fuller and Smith 1980, 1981]. However, several of the physiologic monitoring procedures are invasive (i.e., ingestible plastic thermister used to determine intestinal temperature), socially unacceptable (i.e., rectal thermister), or interfere with communication (i.e., ear thermometer). Physiologic monitoring requires medical supervision and the consent of the worker. See Table 9-1, for examples of physiological monitoring used to prevent heat-related illnesses.
9.4.1 Work and Recovery Heart Rate
One of the earliest procedures for evaluating work and heat strain was introduced by Brouha [1960], in which the body temperature and pulse rate are measured during recovery following a work cycle or at specified times during the workday [Brouha 1960]. At the end of a work cycle, the worker sits on a stool, an oral thermometer is placed under the tongue, and the pulse rate is counted from 30 seconds to 1 minute (P1), from 1.5 to 2 minutes (P2), and from 2.5 to 3 minutes (P3) of seated recovery. If the oral temperature exceeds 37.5°C (99.5°F), the P1 exceeds 110 beats per minutes (bpm), and/or the P1–P3 is fewer than 10 bpm, the heat and work stress is assumed to be above acceptable values. These values are based on group averages and may or may not be protective for an individual worker or specific work situation. However, these values should alert the observer that further review of the job is desirable.
A modified Brouha [1960] approach is being used for monitoring heat stress in some hot industries. An oral temperature and a recovery heart rate pattern have been suggested by Fuller and Smith [1980, 1981] as a basis for monitoring the strain of working at hot jobs. In this modification the primary criterion of high heat strain is an oral temperature exceeding 37.5°C (99.5°F). The heart rate recovery pattern is used to assist in the evaluation. If the P3 is 90 bpm or fewer, the job situation is satisfactory; if the P3 is about 90 bpm and the P1–P3 is about 10 bpm, the pattern indicates that the physical work intensity is high, but there is little if any increase in body temperature; if the P3 is greater than 90 bpm and the P1–P3 is fewer than 10 bpm, the stress (heat + work) is too high for the individual, and corrective actions should be introduced. These individuals should be examined by a responsible healthcare provider, and the work schedule and work environment should be evaluated. The field data reported by Jensen and Dukes-Dobos [1976] corroborate the concept that the P1 recovery heart rate and/or oral temperature are more likely to exceed acceptable values when the environmental plus metabolic heat load exceeds the ACGIH TLVs® for continuous work. The recovery heart rate can be easily measured in workplace situations where being seated for about five minutes will not substantially interfere with the work sequence; in addition, the instrumentation required (a wearable electronic heart rate monitor) can be simple and inexpensive. The recovery and work heart rates can be used on some jobs as early indicators of the strain resulting from heat exposure in hot workplace jobs. The relatively inexpensive, noninvasive electronic devices now available (such as those used by joggers and others) make self-monitoring of work and recovery pulse rates practical for many workplaces.
9.4.2 Body Temperature
The WHO scientific group on Health Factors involved in Working Under Conditions of Heat Stress recommended that the deep body temperature should not, under conditions of prolonged daily work and heat, be permitted to exceed 38°C (100.4°F) or the oral temperature to exceed 37.5°C (99.5°F), although the tolerance to elevated body temperature is quite variable [Taylor et al. 2008]. The limit has generally been accepted by the experts working in the area of workplace heat stress and strain. Given worker permission, body temperature monitoring can be used in many hot jobs.
9.4.2.1 Core Temperature
Monitoring the core body temperature is a direct, objective, and reliable approach. Measuring internal body temperature in the rectum or esophagus may be unacceptable to workers. However, newer technologies, involving an ingestible plastic thermister capable of telemetering “core” (intestinal) temperatures, are in wide use (e.g., CorTemp, HQInc., Palmetto, FL). The disadvantage of the ingestible thermisters that it takes several hours to migrate from the mouth to the small intestine before accurate temperatures can be recorded [Lee et al. 2000; Williams et al. 2011].
It must be noted that tolerance to increased Tre varies widely in individuals. Non-elite runners have been documented to have completed a marathon run with a Tre of >41°C (105.8°F), and a Tre of 41.9°C (107.4°F) has been recorded in soccer players with no adverse physiological consequences [Armstrong et al. 2007b; Taylor et al. 2008]. Therefore, recovery Tre will be different in heat tolerant individuals than in those who are less heat tolerant (see Chapters 5 and 9 for more detailed discussions).
9.4.2.2 Oral Temperature
Oral temperatures are easy to obtain with the inexpensive disposable oral thermometers available. However, to obtain reliable oral temperatures requires a strictly controlled procedure. The thermometer must be correctly placed under the tongue for 3 to 5 minutes before the reading is made, mouth breathing is not permitted during this period, no hot or cold liquids should be consumed for at least 15 minutes beforehand, and the thermometer must not be exposed to an air temperature higher than the oral temperature either before the thermometer has been placed under the tongue or until after the thermometer reading has been taken. In hot environments, this may require that the thermometers be kept in a cool insulated container or immersed in alcohol, except when in the worker’s mouth. Oral temperature is usually lower than deep body temperature by about 0.55°C (0.8°F). With the advent of digital oral thermometers, oral temperatures may be obtained within <30 seconds, thus avoiding some of the issues found with standard alcohol oral thermometers. Evaluation of the significance of any oral temperature must follow established medical and occupational hygiene guidelines.
9.4.2.3 Skin Temperature
The use of skin temperature (Tsk) as a basis for assessing the severity of heat strain and estimating tolerance can be supported by thermodynamically and field-derived data. To move body heat from the deep tissues (core) to the skin (shell), where it is dissipated to the ambient environment, requires an adequate heat gradient. As the skin temperature rises and approaches the core temperature, this temperature gradient is decreased and the rate of heat moved from the core to the shell is decreased and the rate of core heat loss is reduced. To restore the rate of heat loss or core skin heat gradient, the core body temperature would have to increase. Circulation of warm blood from central (core) body compartments to the skin results in an increase in skin temperature. The increase in skin temperature results in heat transfer to the environment through conduction, convection, and radiation as discussed in previous sections of this document. As heat is transferred to the environment, cutaneous blood cools and returns to the body core resulting in a decrease in body core temperature. Under certain environmental conditions, insufficient heat is transferred from the skin to the environment which can result in an increase in core body temperature. As the core temperature is increased above 38°C (100.4°F), the risk of an ensuing heat-related illness is increased.
From these observations, it has been suggested that a reasonable estimate of tolerance time for hot work could be made from the equilibrium lateral thigh or chest skin temperature [Iampietro 1971; Shvartz and Benor 1972; Goldman 1978, 1981, 1985b, 1985a]. Under environmental conditions where evaporative heat exchange is not restricted, skin temperature would not be expected to increase much, if at all. Also, in such situations, the maintenance of an acceptable deep body temperature should not be seriously jeopardized, except under very high metabolic loads or restricted heat transfer. However, when convective and evaporative heat loss is restricted such as when wearing impermeable protective clothing, an estimate of the time required for skin temperature to converge with deep body temperature should provide an acceptable approach for assessing heat strain and for predicting tolerance time. Moreover, although Tsk is generally 2°C to 4°C below body core temperature (Tcore), Tsk can be used to estimate Tcore when other methodologies are not available [Lenhardt and Sessier 2006]. In fact, skin and core temperatures are used as concepts for convenience, because the temperature varies over parts of the body (i.e., both over skin and internally) [Parsons 2003]. One only gives averages of skin or internal temperature. Several authors have attempted to use HR and skin temperature to estimate core temperature, with varying degrees of success. In practice, skin or internal temperatures should be measured directly by techniques discussed elsewhere in this document.
9.4.2.4 Aural Temperature
Aural temperature is measured with an infrared (IR) thermometer in the ear canal. Because the IR thermometer should not come into direct contact with the tympanic membrane, it does not provide a true tympanic temperature. Measurements taken at the peak temperature can be compared with the after-work temperature to compare with the temperature obtained during their work shift to potentially establish work-related hyperthermia. The efficacy of aural temperature measurement to monitor for heat stress is uncertain because it consistently underestimates core temperature, but it is simple and noninvasive [Huggins et al. 2012]. See Table 9-1 for more information on physiological monitoring.
9.4.3 Dehydration
Under heat stress conditions where sweat production may reach 6 to 8 liters in a workday, voluntary replacement of the water lost in the sweat is usually incomplete. The normal thirst mechanism is not sensitive enough to urge us to drink enough water or other fluids to prevent dehydration. If dehydration exceeds 1.5% to 2% of body weight, then tolerance to heat stress begins to deteriorate, heart rate and body temperature increase, and work capacity decreases [Greenleaf and Harrison 1986]. When dehydration exceeds 5%, it may lead to collapse and to dehydration heat-related illness. Since thirst is not an adequate guide for water replacement, workers in hot jobs should be encouraged to drink water or other fluids every 15 to 20 minutes. The fluids should be less than 15°C (59°F). For work that requires an increased level of activity in a hot environment for a prolonged period of time (≥2 hours), sports drinks that contain carbohydrates and electrolytes (e.g., Gatorade) should be used in place of water in order to replace the electrolytes lost from sweating and to avoid hyponatremia (serum sodium concentration <136 mEq·L-1) from excessive consumption of plain water [DOD 2003; Montain and Cheuvront 2008]. Drinking from disposable drinking cups may be preferable to using drinking fountains, as the cups can be taken with the worker and can provide a visual estimate for how much fluid was consumed. The amount of dehydration can be estimated by measuring body weight at intervals during the day or at least at the beginning and end of the work shift. The worker should drink enough water to prevent a loss in body weight. However, because this may not be feasible in all situations, following a recommended water-drinking schedule is usually satisfactory. See 4.1.4.1 Water and Electrolyte Balance and the Influence of Endocrines, for a more complete discussion of recommended hydration strategies.
10| Research Needs
The past decade has brought an enormous increase in our knowledge of heat stress and strain, their relation to health and productivity, techniques and procedures for their assessment, and their health risks. In spite of this, there are several areas where further research can contribute to reducing occupational heat-related health and safety problems.
10.1 Exposure Times and Patterns
In some hot industries, the workers are exposed to heat most of the day; other workers may be exposed only part of the time. Although there is general agreement that there is a relationship between heat stress and the health and safety risks for continuous exposure during an 8-hour workday, there is still uncertainty over acceptable levels of heat stress for intermittent exposure, where the worker may spend only part of the working day in the heat. Issues that need more information include:
- Is a 1-hour, a 2-hour, or an 8-hour TWA required for calculating risk of health effects?
- How long are acceptable exposure times for various total heat loads?
- Are the health effects (heat-related illnesses) and risks the same for intermittent as for continuous heat exposure?
- Do workers exposed intermittently each day to various lengths and amount of heat stress develop heat acclimatization similar to that achieved by continuously exposed workers?
- Are the electrolyte and water bal- ance problems the same for inter- mittently versus continuously heat-exposed workers?
10.2 Deep Body Temperature
The WHO Scientific Group recommended that “it is considered inadvisable for a deep body temperature to exceed 38°C (100.4°F) for prolonged daily exposures (to heat) in heavy work” [WHO 1969] and that a deep body temperature of 39°C (102.2°F) should be considered reason to terminate exposure, even when deep body temperature is being monitored. Issues that need more information include:
- Are these values equally realistic for short-term acute heat exposures as for long-term chronic heat exposures?
- Are these values strongly correlated with increased risk of incurring heat-related illnesses?
- Are these values considered maximal, which are not to be exceeded, mean population levels, or 95th percentile levels?
- Is the rate at which deep body temperature rises to 38°C or 39°C (100.4°F or 102.2°F) important in the health-related significance of the increased body temperature?
- Does a 38°C or 39°C (100.4°F or 102.2°F) deep body temperature have the same health significance if reached after only one hour of exposure as when reached after more than one hour of exposure?
10.3 Electrolyte and Water Balance
The health effects of severe acute negative electrolyte and water balance during heat exposure are well documented. However, the health effects of the imbalances, when derived slowly over periods of months or years, are not known; nor are the effects known for long-term electrolyte loading with and without hyperhydration or hypohydration. An appropriate electrolyte and water regimen for long-term work in the heat requires more data derived from further laboratory and epidemiologic studies.
10.4 Effects of Chronic Heat Exposure
All of the experimental and most of the epidemiologic studies of the health effects of heat stress have been directed toward short exposures of days or weeks and toward acute heat-related illnesses. Little is known about the health consequences of living and working in a hot environment for a working lifetime. Do such long exposures to heat have any morbidity or mortality implications? Does experiencing an acute heat-related illness have any effects on future health and longevity? It is known that individuals with certain health disorders (e.g., diabetes or cardiovascular disease) are less heat tolerant. There is some evidence that the reverse may also be true; for example, chronic heat exposure may render an individual more susceptible to both acute and chronic diseases and disorders [Redmond et al. 1979; Dukes-Dobos 1981]. The chronic effect of heat exposure on blood pressure is a particularly sensitive problem because hypertensive workers may be under treatment with diuretics and on restricted salt diets. Such treatment may be in conflict with the usual emphasis on increased water and salt intake during heat exposure.
10.5 Circadian Rhythm of Heat Tolerance
The normal daily variation in core body temperature from the high point in the mid-afternoon to the low point in the early morning is about 0.5°C [Cheung et al. 2000]. Superimposed on this normal variation in body temperature are increases due to heat exposure. In addition, the WHO report recommends that the 8-hour TWA body temperature of workers in hot industries should not exceed 38°C (100.4°F) [WHO 1969]. Issues that need more information include:
- Is this normal daily increase in body temperature additive to the increase resulting from heat stress?
- Does tolerance to increased body temperature and the connected health risk follow a similar diurnal pattern?
- Would it be necessary to establish different permissible heat exposure limits for day and night shift workers in hot industries?
Also, in addition to the circadian rhythm variations in heat tolerance, there are also rhythmic changes in body temperature and heat tolerance associated with the menstrual cycle [McArdle et al. 2010b]. The effect of the menstrual cycle on core temperature variation (as much a 0.5°C diurnally), particularly as it potentially impacts heat stress and heat tolerance, needs to be better understood.
10.6 Heat Tolerance and Shift Work
It has been estimated that about 30% of workers are on some type of work schedule other than the conventional day work (9 a.m. to 5 p.m.). Shift work, long days with a short week, and double shifts alter the usual living patterns of the worker and result in some degree of sleep deprivation. The effects of these changes in living patterns on heat tolerance are mostly undocumented. Before these changes in work patterns are used, it would be prudent to understand their health and safety implications.
10.7 Detection, Treatment, and Prevention of Heat-related Illnesses
Discovering means of quickly and accurately identifying individuals experiencing or about to experience adverse effects of heat stress exposure—specifically, mechanisms and methods that are inexpensive and that can screen groups of workers quickly—should be a priority. In addition, more could be known about heat cramp etiology and prevention.
Treatment of heat stroke could be improved. A greater understanding of the mechanisms and why, after a certain point, it becomes irreversible could lead to more effective treatments. In addition, are there any medications that might help prevent the body’s inflammatory reactions that often result in death?
10.8 The Effects of Global Climate Change
Global climate change could have a significant effect on indoor and outdoor workers, such as those in agriculture, fishing, construction, and many service areas. In particular, workers located in densely populated areas closer to the equator are at increased risk for heat-related injuries and illnesses, as temperatures are expected to rise in relation to the changing climate and other factors (e.g., low- and middle-income, limited air-conditioning, economic drivers to maintain productivity) [Lucas et al. 2014]. Climate change will not necessarily result in new hazards; however, it may add to the severity, prevalence, and distribution of the already known hazards [Schulte and Chun 2009; Schulte et al. 2015]. Schulte and Chun identify seven categories of climate-related hazards: (1) increased ambient temperature, (2) air pollution, (3) ultraviolet exposure, (4) extreme weather, (5) vector-borne diseases and expanded habitats, (6) industrial transitions and emerging industries, and (7) changes in the built environment. The relationship between these categories and the possible occupational health effect outcomes can be seen in Figure 10-1. In addition, another result of climate change is a reduced work capacity and productivity in heat-exposed jobs, with resulting loss of income, which is also likely to cause mental health and economic effects [Kjellstrom 2009; Kjellstrom et al. 2009b; Berry et al. 2010; Kjellstrom et al. 2010; McMichael 2013].
Adapted from Schulte and Chun [2009].
Figure 10-1. Relationship between climate change and occupational safety and health
How climate change effects on the workforce can be addressed is a relatively new area of research that should be pursued. Some climate change-related risks may be reduced by general improvements in public health, whereas other risks can be managed by ‘adaptation policies and actions’ [Kjellstrom et al. 2009b; Kjellstrom and Weaver 2009; Nilsson and Kjellstrom 2010]. In addition, as relative humidity is expected to remain constant in a changing climate, WBGT-based occupational exposure limits may remain unchanged based on this factor [Ingram 2002]. The idea to develop a program to capture the growing evidence on climate change and health emerged at a 1998 Intergovernmental Panel on Climate Change meeting, and it was eventually presented as the ‘high occupational temperature health and productivity suppression’ (Hothaps) program [Kjellstrom et al. 2009a]. The Hothaps program is a multi-center health research and prevention program used to quantify the extent to which workers are affected by, or adapt to, heat exposure while working, and how global heating during climate change may increase such effects. Programs like Hothaps and others will help to capture current heat-related events, likely leading to new heat-related occupational safety and health recommendations and regulations in the future.
10.9 Heat Stress and Toxicology
Exposure to heat can affect how chemicals are absorbed into the body. Since the 1890s, animal studies have shown that exposure to heat exacerbates chemical absorption and toxicity [Leon 2008]. Leon states that changes to the body’s core temperature can alter absorption, distribution, metabolism, and excretion of the toxicants. Increases in respiration can lead to further toxicant exposure through inhalation, whereas increases in sweat and skin blood flow can lead to more efficient transcutaneous absorption of some toxicants [Gordon 2003; Leon 2008]. The relationships between how heat and other factors can affect the physiological response to toxicants can be seen in Figure 10-2.
Adapted from Gordon [2003].
Figure 10-2. How heat, humidity, work, and thermoregulation affect the physiological response to toxicants
Animal studies examining thermal stress and the effects on chemical toxicity show that heat plays a role in toxicant absorption, but are difficult to interpret when trying to extrapolate to humans. Test animals tend to be sedentary with no option for exercise, are acclimatized to ideal environmental conditions, and use hypothermia as their predominant thermoregulatory response to chemical toxicants [Gordon 2003; Leon 2008]. In vitro and in vivo studies have suggested that heat stress, with or without exercise, will activate thermoeffectors (e.g., skin blood flow, sweating, respiration) that, in turn, accelerate pesticide absorption in humans [Gordon and Leon 2005]. Gordon and Leon [2005] also mention an in vitro model used to show blood flow, temperature, and RH and the effect on absorption of the pesticide parathion, as well as human studies showing the accelerating effects of perspiration on the absorption of organophosphorus compounds. Pesticides are also a hazard to workers in the heat, as high temperatures accelerate dispersion, increase the density of airborne particles, and some workers remove their PPE due to discomfort in the heat [Gordon 2003]. The following may be at risk of heat stress in the presence of chemicals: farmers and other agricultural workers, food-processing laborers, construction workers, fire fighters, and foundry and manufacturing workers [Bourbonnais et al. 2013].
Most of what is known about toxicants is derived from animal studies in which the animals were kept in comfortable temperatures; therefore, a better understanding of the mechanisms involved between heat exposure and toxicants in humans is needed [Gordon 2003; Gordon and Leon 2005]. With changes in the climate and hotter temperatures, the need for more information on toxicants and their relationship to heat stress will become increasingly important [Leon 2008].
10.10 Other Research Needs
Other suggested research needs include validity/sensitivity and specificity of personal monitoring methods, effective management methods (e.g., value and content of training), and epidemiological studies to evaluate the RAL and REL where outcomes can be heat-related disorders, productivity, and injuries [Bernard 2014]. In addition, research is needed on the development of more effective and cheaper PPE to protect workers against heat illness and injury. These activities are ongoing, and the future will no doubt bring new technologies to mitigate the effects of heat on workers and reduce the incidence of heat-induced injury, illness, and death.
References
ACGIH [1985]. TLVs®, threshold limit values for chemical substances and physical agents in the work environment and biological exposure indices with intended changes for 1985–86. Cincinnati, OH: American Conference of Governmental Industrial Hygienists.
ACGIH [2014]. TLVs® and BEIs®: threshold limit values for chemical substances and physical agents and biological exposure indices. Cincinnati, OH: American Conference of Governmental Industrial Hygienists.
AIHA [1971]. Ergonomics guide to assessment of metabolic and cardiac costs of physical work. Am Ind Hyg Assoc J 32(8):560–564.
AIHA [2003]. The occupational environment: its evaluation, control, and management. 2nd ed. Fairfax, VA: AIHA Press.
Ainsworth BE, Haskell WL, Herrmann SD, Meckes N, Bassett DR Jr, Tudor-Locke C, Greer JL, Vezina J, Whitt-Glover MC, Leon AS [2011]. 2011 Compendium of physical activities: a second update of codes and MET values. Med Sci Sports Exerc 43(8):1575–1581.
Allen RW, Ells MD, Hart AW [1976]. Industrial hygiene. Englewood Cliffs, NJ: Prentice-Hall.
Almond CS, Shin AY, Fortescue EB, Mannix RC, Wypij D, Binstadt BA, Duncan CN, Olson DP, Salerno AE, Newburger JW, Greenes DS [2005]. Hyponatremia among runners in the Boston marathon. N Engl J Med 352(15):1550–1556.
Alpers JP, Jones LK Jr [2010]. Natural history of exertional rhabdomyolysis: a population-based analysis. Muscle Nerve 42(4):487–491.
Arbury S, Jacklitsch B, Farquah O, Hodgson M, Lamson G, Martin H, Profitt A, Office of Occupational Health Nursing OSHA [2014]. Heat illness and death among workers: United States, 2012–2013. MMWR Morb Mortal Wkly Rep 63(31):661–665.
Armed Forces Health Surveillance Center [2011]. Surveillance snapshot: reportable medical events of heat injury in relation to heat index, June–September 2011. MSMR 18(10):19.
Armed Forces Health Surveillance Center [2014]. Update: exertional rhabdomyolysis, active component, US Armed Forces, 2009–2013. MSMR 21(3):14.
Armstrong CG, Kenney WL [1993]. Effects of age and acclimation on responses to passive heat exposure. J Appl Physiol 75(5):2162–2167.
Armstrong L, Pumerantz A, Fiala K, Roti M, Kavouras S, Casa D, Maresh C [2010]. Human hydration indices: acute and longitudinal reference values. Intl J Sport Nutrit Exercise Metab 20(2):145–153.
Armstrong LE, Casa DJ, Maresh CM, Ganio MS [2007a]. Caffeine, fluid-electrolyte balance, temperature regulation, and exercise-heat tolerance. Exerc Sport Sci Rev 35(3):135–140.
Armstrong LE, Casa DJ, Millard-Stafford M, Moran DS, Pyne SW, Roberts WO [2007b]. American College of Sports Medicine position stand: exertional heat illness during training and competition. Med Sci Sports Exerc 39(3):556–572.
Armstrong LE, De Luca JP, Hubbard RW [1990]. Time course of recovery and heat acclimation ability of prior exertional heatstroke patients. Med Sci Sports Exerc 22(1):36–48.
Armstrong LE, Maresh CM, Castellani JW, Bergeron MF, Kenefick RW, LaGasse KE, Riebe D [1994]. Urinary indices of hydration status. Int J Sport Nutr 4(3):265–279.
Armstrong LE, Soto JA, Hacker FT Jr, Casa DJ, Kavouras SA, Maresh CM [1998]. Urinary indices during dehydration, exercise, and rehydration. Int J Sport Nutr 8(4):345–355.
Armstrong LE, Stoppani J [2002]. Central nervous system control of heat acclimation adaptations: an emerging paradigm. Rev Neurosci 13(3):271–285.
ASHRAE [1981a]. ASHRAE handbook: 1981 fundamentals. Atlanta: The American Society of Heat, Refrigeration, and Air Conditioning Engineers.
ASHRAE [1981b]. Physiological principles, comfort, and health. In: ASHRAE handbook: 1981 fundamentals. Atlanta: The American Society of Heat, Refrigeration, and Air Conditioning Engineers.
Åstrand P-O, Rodahl K [1977]. Textbook of work physiology: physiological bases of exercise. 2nd ed. New York: McGraw-Hill.
Åstrand P-O, Rodahl K, Dahl HA, Strømme SB [2003]. Textbook of work physiology. Champaign, IL: Human Kinetics.
Avellini BA, Kamon E, Krajewski JT [1980a]. Physiological responses of physically fit men and women to acclimation to humid heat. J Appl Physiol 49(2):254–261.
Avellini BA, Shapiro Y, Pandolf KB, Pimental NA, Goldman RF [1980b]. Physiological responses of men and women to prolonged dry heat exposure. Aviat Space Environ Med 51(10):1081–1085.
Banister EW, Brown SR [1968]. The relative energy requirements of physical activity, exercise physiology. New York: Academic Press.
Bar-Or O, Lundegren HM, Buskirk ER [1969]. Heat tolerance of exercising obese and lean women. J Appl Physiol 26(4):403–409.
Belding HS [1971]. Evaluation of stresses of exposure to heat. Pittsburgh: University of Pittsburgh.
Belding HS [1973]. Control of exposures to heat and cold. In: National Institute for Occupational Safety and Health, United States Public Health Service, Division of Occupational Health, ed. The industrial environment: its evaluation & control. 3rd ed. Washington, DC: U. S. Government Printing Office.
Belding HS, Hatch TF [1955]. Index for evaluating heat stress in terms of reslting physiological strain. Heat Pip Air Condit 27:129–135.
Benedict RF [1977]. Fundamentals of temperature, pressure and flow measurements. New York: John Wiley and Sons.
Berger RA [1982]. Applied exercise physiology. Philadelphia, PA: Lea & Febiger.
Berko J, Ingram DD, Saha S, Parker JD [2014]. Deaths attributed to heat, cold, and other weather events in the United States, 2006–2010. Natl Health Stat Report (76):1–15.
Bernard TE [2014]. Occupational heat stress in USA: whither we go? Ind Health 52(1):1–4.
Bernard TE, Ashley CD [2009]. Short-term heat stress exposure limits based on wet bulb globe temperature adjusted for clothing and metabolic rate. J Occup Environ Hyg 6(10):632–638.
Bernard TE, Barrow CA [2013]. Empirical approach to outdoor WBGT from meteorological data and performance of two different instrument designs. Ind Health 51(1):79–85.
Bernard TE, Kenney WL [1994]. Rationale for a personal monitor for heat strain. Am Ind Hyg Assoc J 55(6):505–514.
Bernard TE, Pourmoghani M [1999]. Prediction of workplace wet bulb global temperature. Appl Occup Environ Hyg 14(2):126–134.
Berry HL, Bowen K, Kjellstrom T [2010]. Climate change and mental health: a causal pathways framework. Int J Public Health 55(2):123–132.
Beshir MY [1981]. A comprehensive comparison between WBGT and Botsball. Am Ind Hyg Assoc J 42:81–87.
Beshir MY, Ramsey JD, Burford CL [1982]. Threshold values for Botsball: a field study of ergonomics. Ergonomics 25:247–254.
Blazejczyk K, Jendritzky G, Bröde P, Fiala D, Havenith G, Epstein Y, Psikuta A, Kampmann B [2013]. An introduction to the Universal Thermal Climate Index (UTCI). Geographia Polonica 86(1):5–10.
BLS [2011]. Occupational outlook handbook, 2010–2011. Washington, DC: U.S. Department of Labor, Bureau of Labor Statistics.
Bosch X, Poch E, Grau JM [2009]. Rhabdomyolysis and acute kidney injury. N Engl J Med 361(1):62–72.
Botsford JH [1971]. A wet globe thermometer for environmental heat measurement. Am Ind Hyg Assoc J 32(1):1–10.
Bourbonnais R, Zayed J, Levesque M, Busque MA, Duguay P, Truchon G [2013]. Identification of workers exposed concomitantly to heat stress and chemicals. Ind Health 51(1):25–33.
Brouha L [1960]. Physiology in industry: evaluation of industrial stresses by the physiological reactions of the worker. New York: Pergamon Press.
Brudvig TJ, Fitzgerald PI [2007]. Identification of signs and symptoms of acute exertional rhabdomyolysis in athletes: a guide for the practitioner. Strength Condit J 29(1):10–14.
Burke LM [2008]. Sports supplements debate: a risky practice that produces expensive urine or legitimate performance boosts that can be found in a acket or bottle? In: Taylor NAS, Groeller H, eds. Physiological bases for human performance during work and exercise. Edinburgh: Churchhill Livingstone Elsevier.
Buskirk ER, Bass DE [1980]. Climate and exercise. In: Johnson WR, Buskirk ER, eds. Structural and physiological aspects of exercise and sport. Princeton, NJ: Princeton Book Co.
Cal/OSHA [2010]. Heat illness prevention campaign: final performance and evaluation report, http://www.dir.ca.gov/DOSH/...
Canadian Centre for Occupational Health and Safety [2011]. Thermal comfort for office work, http://www.ccohs.ca/oshanswers/phys_agents/thermal_comfort.html.
Candas V, Libert JP, Vogt JJ [1979]. Influence of air velocity and heat acclimation on human skin wettedness and sweating efficiency. J Appl Physiol 47(6):1194–1200.
Casa DJ, Csillan D, Armstrong LE, Baker LB, Bergeron MF, Buchanan VM, Carroll MJ, Cleary MA, Eichner ER, Ferrara MS, Fitzpatrick TD, Hoffman JR, Kenefick RW, Klossner DA, Knight JC, Lennon SA, Lopez RM, Matava MJ, O’Connor FG, Peterson BC, Rice SG, Robinson BK, Shriner RJ, West MS, Yeargin SW [2009]. Preseason heat-acclimatization guidelines for secondary school athletics. J Athl Train 44(3):332–333.
CDC [1990]. Exertional rhabdomyolysis and acute renal impairment: New York City and Massachusetts, 1988. MMWR Morb Mortal Wkly Rep 39(42):751.
CDC [2008]. Heat-related deaths among crop workers: United States, 1992–2006. MMWR Morb Mortal Wkly Rep 57(24):649–653.
CDC [2009]. Extreme heat: a prevention guide to promote your personal health and safety, http://www.cdc.gov/disasters/extremeheat/index.html.
Cena K, Clark JA, Politechnika Wroclawska [1981]. Bioengineering, thermal physiology, and comfort. Amsterdam: Elsevier Scientific Publishing Co.; New York: Elsevier/North-Holland, distributor; p. 289.
Cervellin G, Comelli I, Lippi G [2010]. Rhabdomyolysis: historical background, clinical, diagnostic and therapeutic features. Clin Chem Lab Med 48(6):749–756.
Chang SX, Ge XS [1983]. Preliminary research in the measurement of the solar radiation by transient technique. Solar Energy 30:391–395.
Chatzizisis YS, Koskinas KC, Misirli G, Vaklavas C, Hatzitolios A, Giannoglou GD [2010]. Risk factors and drug interactions predisposing to statin-induced myopathy: implications for risk assessment, prevention and treatment. Drug Safety 33(3):171–187.
Chen ML, Chen CJ, Yeh WY, Huang JW, Mao IF [2003]. Heat stress evaluation and worker fatigue in a steel plant. AIHA J 64(3):352–359.
Cheung SS, McLellan TM, Tenaglia S [2000]. The thermophysiology of uncompensable heat stress: physiological manipulations and individual characteristics. Sports Med 29(5):329–359.
Chung NK, Pin CH [1996]. Obesity and the occurrence of heat disorders. Mil Med 161(12):739–742.
Ciricello VM, Snook SH [1977]. The prediction of WBGT from Botsball. Am Ind Hyg Assoc J 38:264–271.
Clapp JF 3rd [1991]. The changing thermal response to endurance exercise during pregnancy. Am J Obstet Gynecol 165(6 Pt 1):1684–1689.
Clarren SK, Smith DW, Harvey MA, Ward RH, Myrianthopoulos NC [1979]. Hyperthermia: a prospective evaluation of a possible teratogenic agent in man [research support: US Public Health Service]. J Pediatr 95(1):81–83.
Coca A, Roberge RJ, Williams WJ, Landsittel DP, Powell JB, Palmiero A [2010]. Physiological monitoring in fire fighter ensembles: wearable plethysmographic sensor vest versus standard equipment. J Occup Environ Hyg 7(2):109–114.
Coolerado Corporation [2012]. Psychrometric charts. Denver: Coolerado, http://www.coolerado.com/pdfs/Coolerado-Psychrometric-Comparison.pdf.
Cooper KE, Veale WL, Kasting NW [1982]. Temperature regulation, fever and antipyreics. In: Barnett HJM, ed. New uses for old drugs. New York: Raven Press.
Counselman FL, Lo BM [2011]. Chapter 92: rhabdomyolysis. In: Tintinalli JE, Stapczynski JS, Ma OJ, Cline DM, Cydulka RK, Meckler GD, and Physicians TACoE, eds. Tintinalli’s emergency medicine: a comprehensive study guide. 7th ed. New York: The McGraw-Hill Companies.
Curriero FC, Heiner KS, Samet JM, Zeger SL, Strug L, Patz JA [2002]. Temperature and mortality in 11 cities of the eastern United States. Am J Epidemiol 155(1):80–87.
Dasler AR [1977]. Heat stress, work function and physiological heat exposure limits in man. Gaithersburg, MD: National Bureau of Standards.
Davies CTM, Brotherhood JR, Collins KJ, Doré C, Imms F, Musgrove J, Weiner JS, Amin MA, Ismail HM, El Karim M, Omer AHS, Sukkar MY [1976]. Energy expenditure and physiological performance of Sudanese cane cutters. Br J Indust Med 33:181–186.
de Carvalho JF, da Mota LM, Bonfa E [2011]. Fatal rhabdomyolysis in systemic lupus erythematosus. Rheumatol Int 31(9):1243–1245.
Dehoney S, Wellein M [2009]. Rhabdomyolysis associated with the nutritional supplement hydroxycut. Am J Health Syst Pharm 66(2):142–148.
DiBenedetto JP, Worobec SM [1985]. Exposure to hot environments can cause dermatological problems. Occup Health Saf 54:35–38.
Dinman BD, Horvath SM [1984]. Heat disorders in industry: a reevaluation of diagnostic criteria. J Occup Med 26(7):489–495.
Do KD, Bellabarba C, Bhananker SM [2007]. Exertional rhabdomyolysis in a bodybuilder following overexertion: a possible link to creatine overconsumption. Clin J Sport Med 17(1):78–79.
DOD [1980]. Occupational and environmental health: prevention, treatment, and control of heat injury. Washington, DC: Departments of the U.S. Army, Navy, and Air Force.
DOD [2003]. Technical bulletin: heat stress control and heat casualty management. TB MED 507/AFPAM 48-152 (I). Washington, DC: Departments of the U.S. Army, Navy, and Air Force.
Drinkwater BL, Denton JE, Kupprat IC, Talag TS, Horvath SM [1976]. Aerobic power as a factor in women’s response to work in hot environments. J Appl Physiol 41(6):815–821.
Drinkwater BL, Horvath SM [1979]. Heat tolerance and aging. Med Sci Sports 11(1):49–55.
Duffie JA, Beckman WA [1980]. Solar engineering of thermal processes. New York: Wiley.
Dukes-Dobos FN [1981]. Hazards of heat exposure: a review. Scand J Work Environ Health 7(2):73–83.
Dukes-Dobos FN, Henschel A [1973]. Development of permissible heat exposure limits for occupational work. ASHRAE, Journal of the American Society of Heating Refrigerating and Air-Conditioning Engineers 57–62.
Edwards MJ [2006]. Review: hyperthermia and fever during pregnancy. Birth Defects Res A Clin Mol Teratol 76(7):507–516.
Ellis FP [1972]. Mortality from heat illness and heat-aggravated illness in the United States. Environ Res 5(1):1–58.
Ely BR, Ely MR, Cheuvront SN [2011]. Marginal effects of a large caffeine dose on heat balance during exercise-heat stress. Int J Sport Nutr Exerc Metab 21(1):65–70.
EPA [1993]. A guide to heat stress in agriculture. EPA-750-b-92-001. Washington, DC: US Environmental Protection Agency.
Epstein Y, Albukrek D, Kalmovitc B, Moran DS, Shapiro Y [1997]. Heat intolerance induced by antidepressants [case report]. Ann NY Acad Sci 813:553–558.
Food and Nutrition Board, Institute of Medicine [2004]. Dietary reference intakes for water, potassium, sodium, chloride, and sulfate. Washington, DC: The National Academies Press.
Frye AJ, Kamon E [1981]. Responses to dry heat of men and women with similar aerobic capacities. J Appl Physiol 50(1):65–70.
Fuller FH, Smith PE Jr [1980]. The effectiveness of preventive work practices in a hot workshop. No. 81-108. Presented at the NIOSH Workshop on Recommended Heat Stress Standards, Cincinnati, OH.
Fuller FH, Smith PE Jr [1981]. Evaluation of heat stress in a hot workshop by physiological measurements. Am Ind Hyg Assoc J 42(1):32–37.
Gagge AP [1970]. Effects of radiant flux, an independent variable that describes thermal radiation on man/physiological and behavioral temperature regulation. Springfield, IL: Charles C Thomas.
Gagnon D, Kenny GP [2011]. Sex modulates whole-body sudomotor thermosensitivity during exercise. J Physiol 589(Pt 24):6205–6217.
Gardner JW, Kark JA [1994]. Fatal rhabdomyolysis presenting as mild heat illness in military training. Military Med 159(2):160–163.
Garg A [1982]. Treatise on solar energy. In: Fundamentals of solar energy. Vol. 1. New York: John Wiley and Sons.
Garg A, Chaffin DB, Herrin GD [1978]. Prediction of metabolic rates for manual materials handling jobs. Am Ind Hyg Assoc J 39(8):661–674.
George M, Delgaudio A, Salhanick SD [2010]. Exertional rhabdomyolysis: when should we start worrying? Case reports and literature review. Pediatr Emerg Care 26(11):864–866.
Gisolfi CV [2000]. Is the GI system built for exercise? News Physiol Sci 15:114–119.
Givoni B, Rim Y [1962]. Effect of the thermal environment and psychological factors upon subjects’ responses and performance of mental work. Ergonomics 5:99–119.
Golden JS, Hartz D, Brazel A, Luber G, Phelan P [2008]. A biometeorology study of climate and heat-related morbidity in Phoenix from 2001 to 2006. Int J Biometeorol 52(6):471–480.
Goldman RF [1973]. Clothing, its physiological effects, adequacy in extreme thermal environments, and possibility of future improvements. Arch Sci Physiol (Paris) 27(2):137–147.
Goldman RF [1978]. Prediction of human heat tolerance. In: Folinsbee LJ, ed. Environmental stress: individual human adaptations. New York: Academic Press.
Goldman RF [1981]. Evaluating the effects of clothing on the wearer. In: Cena K, Clark JA, Politechnika Wrocawska, eds. Bioengineering, thermal physiology, and comfort. Amsterdam: Elsevier Scientific Publishing Co.; New York: Elsevier/North-Holland, distributor.
Goldman RF [1985a]. Heat stress in industrial protective encapsulating garments. In: Levine SP, Martin WF, eds. Protecting Personnel at Hazardous Waste Sites. Vol. 10. Boston: Butterworth Publishers.
Goldman RF [1985b]. Personal protective equipment. In: Levine SP, Martin WF, eds. Protecting personnel at hazardous waste sites. Vol. 10. Boston: Butterworth Publishers.
González-Alonzo J, Eiken O, Mekjavic IB [2008]. A critical core temperature and the significance of absolute work rate. In: Taylor NAS, Groeller H, eds. The physiological bases of human performance during work and exercise. Edinburgh: Churchill Livingstone Elsevier.
Gonzalez RR, Berglund LG, Gagge AP [1978]. Indices of thermoregulatory strain for moderate exercise in the heat. J Appl Physiol 44(6):889–899.
Gordon CJ [2003]. Role of environmental stress in the physiological response to chemical toxicants. Environ Res 92(1):1–7.
Gordon CJ, Leon LR [2005]. Thermal stress and the physiological response to environmental toxicants. Rev Environ Health 20(4):235–263.
Grayson J, Kuehn LA [1979]. Heat transfer and heat loss. In: Lomax P, ed. Body temperature, regulation, drug effects, and therapeutic implications. New York: Dekker.
Greenleaf JE [1979]. Hyperthermia and exercise. Intl Rev Physiol 20:157–208.
Greenleaf JE, Harrison MH [1986]. Water and electrolytes. ACS Symp Series 294:107–124.
Haller PR [2011]. Compartment syndrome. In: Tintinalli JE, Stapczynski JS, Ma OJ, Cline DM, Cydulka RK, Meckler GD, Physicians TACoE, eds. Tintinalli’s emergency medicine: a comprehensive study guide. 7th ed. New York: The McGraw-Hill Companies.
Hancock PA [1981]. Heat stress impairment of mental performance: a revision of tolerance limits. Aviat Space Environ Med 52(3):177–180.
Hancock PA [1982]. Task categorization and the limits of human performance in extreme heat. Aviat Space Environ Med 53(8):778–784.
Havenith G [1999]. Heat balance when wearing protective clothing. Ann Occup Hyg 43(5):289–296.
Heat Stress Management Program for the Nuclear Power Industry: Interim Report [1986]. Report prepared for the Electric Power Research Institute, Palo Alto, CA.
Hellon RF, Lind AR [1958]. The influence of age on peripheral vasodilatation in a hot environment. J Physiol 141(2):262–272.
Henderson J, Baker HW, Hanna PJ [1986]. Occupation-related male infertility: a review. Clin Reprod Fertil 4(2):87–106.
Henschel A [1967]. Obesity as an occupational hazard. Can J Public Health 58(11):491–493.
Henschel A [1971]. The environment and performance. In: Simonson E, ed. Physiology of work capacity and fatigue. Vol. 14. Springfield, IL: Charles C Thomas.
Henschel A, Burton LL, Margolies L, Smith JE [1969]. An analysis of the heat deaths in St. Louis during July, 1966. Am J Public Health Nations Health 59(12):2232–2242.
Herman RM, Brower JB, Stoddard DG, Casano AR, Targovnik JH, Herman JH, Tearse P [2007]. Prevalence of somatic small fiber neuropathy in obesity. Int J Obes (Lond) 31(2):226–235.
Horvath SM [1985]. Hot and cold environments. In: Cralley and Cralley, eds. Patty’s industrial hygiene and toxicology. 2nd ed.
HSDB [2011]. Caffeine (CAS No. 58-08-2). Hazardous Substance Databank. Huerta-Alardin AL, Varon J, Marik PE [2005]. Bench-to-bedside review: rhabdomyolysis. An overview for clinicians. Crit Care 9(2):158–169.
Huggins R, Glaviano N, Negishi N, Casa DJ, Hertel J [2012]. Comparison of rectal and aural core body temperature thermometry in hyperthermic, exercising individuals: a meta-analysis. J Athl Train 47(3):329–338.
Iampietro PF [1971]. Use of skin temperature to predict tolerance to thermal environments. Aerosp Med 42:396–399.
Inbar O, Morris N, Epstein Y, Gass G [2004]. Comparison of thermoregulatory responses to exercise in dry heat among prepubertal boys, young adults and older males. Exp Physiol 89(6):691–700.
Ingram WJ [2002]. On the robustness of the water vapor feedback: gcm vertical resolution and formulation. J Clim 15(9):917–921.
Inoue Y, Havenith G, Kenney WL, Loomis JL, Buskirk ER [1999]. Exercise- and methylcholine-induced sweating responses in older and younger men: effect of heat acclimation and aerobic fitness. Int J Biometeorol 42(4):210–216.
ISO [1982a]. Hot environments: estimation of heat stress on working man based on the WBGT index (ISO 7243). Geneva: ISO.
ISO [1982b]. Thermal environments: analytical determination of thermal stress (ISO/DP7933). Geneva: ISO.
ISO [1989]. Hot environments: estimation of heat stress on working man based on the WBGT Index (ISO 7243). Geneva: ISO.
ISO [1990]. determination of metabolic rate (ISO 8996). Geneva: ISO.
ISO [1993]. Evaluation of cold environments: determination of required clothing insulation (IREQ) (ISO TR 11079). Geneva: ISO.
ISO [2004a]. Ergonomics: evaluation of thermal strain by physiological measurements (Standard No. ISO 9886). Geneva: ISO.
ISO [2004b]. Ergonomics of the thermal environment: analytical determination and interpretation of heat stress using calculation of the predicted heat strain (Standard No. ISO 7933). Geneva: ISO.
ISO [2004c]. Ergonomics of the thermal environment: determination of metabolic rate (Standard No. ISO 8996). Geneva: ISO.
ISO [2007]. Ergonomics of the thermal environment: estimation of thermal insulation and water vapour resistance of a clothing ensemble (Standard No. ISO 9920). Geneva: ISO.
ISO [2012]. International organization for standardization: home page, http://www.iso.org/iso/home.htm. Geneva: ISO.
Jackson EK [2006]. Renin and angiotensin. In: Brunton LL, Lazo JS, Parker KL, eds. Goodman & Gilman’s The pharmacologic basis of therapeutics. 11th ed. New York: McGraw-Hill.
Japan Society for Occupational Health [2005]. Recommendation of occupational exposure limits 2005–2006. J Occup Health 47:354–370.
Jensen RC, Dukes-Dobos FN [1976]. Validation of proposed limits for exposure to industrial heat. Presented at the Standards of Occupational Exposure to Hot Environments conference.
Johnson AT, Kirk GD [1980]. Correlation of WBGT and Botsball sensors. Am Ind Hyg Assoc J 41(5):361–366.
Joy RJ, Goldman RF [1968]. A method of relating physiology and military performance: a study of some effects of vapor barrier clothing in a hot climate. Military Med 133(6):458–470.
Jung A, Schuppe HC [2007]. Influence of genital heat stress on semen quality in humans. [review]. Andrologia 39(6):203–215.
Kamon E, Avellini B [1979]. Wind speed limits to work under hot environments for clothed men. J Appl Physiol 46(2):340–345.
Kamon E, Ryan C [1981]. Effective heat strain index using pocket computer. Am Ind Hyg Assoc J 42(8):611–615.
Kandel E, Schwartz J [2013]. Principles of neural science. 5th ed. New York: McGraw-Hill Education.
Karpovich PV, Sinning WE [1971]. Physiology of muscular activity. Philadelphia: Saunders.
Kenefick RW, Cheuvront SN, Palombo LF, Ely BR, Sawka MS [2010]. Skin temperature modifies the impact of hypohydration on aerobic performance. J Appl Physiol 109:79–86.
Kenney WL, Tankersley CG, Newswanger DL, Hyde DE, Puhl SM, Turner NL [1990]. Age and hypohydration independently influence the peripheral vascular response to heat stress. J Appl Physiol 68(5):1902–1908.
Kenny GP, Webb P, Ducharme MB, Reardon FD, Jay O [2008]. Calorimetric measurement of postexercise net heat loss and residual heat storage. Med Sci Sports Exerc 40(9):1629–1636.
Kenny GP, Yardley J, Brown C, Sigal RJ, Jay O [2010]. Heat stress in older individuals and patients with common chronic diseases. CMAJ 182(10):1053–1060.
Kerslake DM [1972]. The stress of hot environments. Cambridge: University Press.
Khagali M, Hayes JSR [1983]. Heatstroke and temperature regulation. Sydney: Academic Press.
Khan FY [2009]. Rhabdomyolysis: a review of the literature. Neth J Med 67(9):272–283.
Khogali M [1983]. Epidemiology of heat illnesses during the Makkah Pilgrimages in Saudi Arabia. Int J Epidemiol 12(3):267–273.
Khogali M [1997]. Heat illness alert program: practical implications for management and prevention. Ann NY Acad Sci 813:526–533.
Kilbourne EM, Choi K, Jones TS, Thacker SB [1982]. Risk factors for heatstroke: a case-control study. JAMA 247(24):3332–3336.
Kim JH, Coca A, Williams WJ, Roberge RJ [2011]. Effects of liquid cooling garments on recovery and performance time in individuals performing strenuous work wearing a fire fighter ensemble. J Occup Environ Hyg 8(7):409–416.
Kirk PM, Sullman MJM [2001]. Heart rate strain in cable hauler choker setters in New Zealand logging operations. Appl Ergonomics 32:389–398.
Kjellstrom T [2009]. Climate change, direct heat exposure, health and well-being in low and middle-income countries. Glob Health Action 2.
Kjellstrom T, Butler AJ, Lucas RM, Bonita R [2010]. Public health impact of global heating due to climate change: potential effects on chronic non-communicable diseases. Int J Public Health 55(2):97–103.
Kjellstrom T, Gabrysch S, Lemke B, Dear K [2009a]. The ‘Hothaps’ programme for assessing climate change impacts on occupational health and productivity: an invitation to carry out field studies. Glob Health Action 2.
Kjellstrom T, Holmer I, Lemke B [2009b]. Workplace heat stress, health and productivity: an increasing challenge for low and middle-income countries during climate change. Glob Health Action 2.
Kjellstrom T, Weaver HJ [2009]. Climate change and health: impacts, vulnerability, adaptation and mitigation. NSW Public Health Bull 20(1–2):5–9.
Knochel JP [1974]. Environmental heat illness: an eclectic review. Arch Intern Med 133(5):841–864.
Knowlton K, Lynn B, Goldberg RA, Rosenzweig C, Hogrefe C, Rosenthal JK, Kinney PL [2007]. Projecting heat-related mortality impacts under a changing climate in the New York City region. Am J Public Health 97(11):2028–2034.
Kuehn LA [1973]. Response of the globe temperature. Canada: Department of National Defense, OCHIEM, N.859. Kuehn LA, Machattie LE [1975]. A fast responding and direct reading WBGT index meter. AIHA J 36:325–331.
Kuennen M, Gillum T, Dokladny K, Bedrick E, Schneider S, Moseley P [2011]. Thermotolerance and heat acclimation may share a common mechanism in humans. Am J Physiol Regul Integr Comp Physiol 301(2):R524–R533.
Lary JM [1984]. Hyperthermia and teratogenicity. In: Anghileri LJ, Robert J, eds. Hyperthermia in cancer treatment. Boca Raton, FL: CRC Press.
Lee DH, Henschel A [1963]. Evaluation of thermal environment in shelters (No. TR-8). Washington, DC: U.S. Public Health Service, Division of Occupational Health.
Lee SMC, Williams WJ, Fortney-Schneider [2000]. Core temperature measurement during supine exercise: esophageal, rectal, and intestinal temperatures. Aviat Space Environ Med 71(9):939–945.
Leithead CS, Lind AR [1964]. Heat stress and heat disorders. London: Cassell.
Lenhardt R, Sessier DI [2006]. Estimation of mean-body temperature from mean skin and core temperature. Anesthesiology 105(6):1117–1121.
Leon LR [2007]. Heat stroke and cytokines. Prog Brain Res 162:481–524.
Leon LR [2008]. Thermoregulatory responses to environmental toxicants: the interaction of thermal stress and toxicant exposure. Toxicol Appl Pharmacol 233(1):146–161.
Levine RJ [1984]. Male fertility in hot environment. JAMA 252(23):3250–3251.
Lind AR [1976]. Limits of exposure to work in hot climates without a rise in body temperature. In: Horvath SM, Jensen RC, eds. Standards for occupational exposures to hot environments. Publ. No. 76-100. Vol. 3. U.S. Department of Health, Education, and Welfare, National Institute for Occupational Safety and Health.
Lind AR [1977]. Human tolerance to hot climates. In: Lee DHK, Falk HL, Murphy SD, American Physiological Society, eds. Handbook of physiology: a critical, comprehensive presentation of physiological knowledge and concepts. Bethesda, MD: American Physiological Society.
Lind AR [1970]. Effect of individual variation on upper limit of prescriptive zone of climates. J Appl Physiol 28(1):57–62.
Lind AR. [1963] A physiological criterion for setting thermal environmental limits for everyday work. J Appl Physiol 18(1):51–6.
Lind AR, Bass DE [1963]. Optimal exposure time for development of acclimatization to heat. Fed Proc 22:704–708.
Lind AR, Humphreys PW, Collins KJ, Foster K, Sweetland KF [1970]. Influence of age and daily duration of exposure on responses of men to work in heat. J Appl Physiol 28(1):50–56.
Lindqvist PG, Marsal K, Merlo J, Pirhonen JP [2003]. Thermal response to submaximal exercise before, during and after pregnancy: a longitudinal study. J Matern Fetal Neonatal Med 13(3):152–156.
Line RL, Rust GS [1995]. Acute exertional rhabdomyolysis. Am Fam Phys 52(2):502–506.
Logan PW, Bernard TE [1999]. Heat stress and strain in an aluminum smelter. Am Ind Hyg Assoc J 60(5):659–665.
Luber G, McGeehin M [2008]. Climate change and extreme heat events. Am J Prev Med 35(5):429–435.
Lucas RA, Epstein Y, Kjellstrom T [2014]. Excessive occupational heat exposure: a significant ergonomic challenge and health risk for current and future workers. Extrem Physiol Med 3:14.
Luginbuhl RC, Castillo DN, Loringer KA [2008]. Heat-related deaths among crop workers: United States, 1992–2006. MMWR Morb Mortal Wkly Rep 57(24):649–653.
Macpherson RK [1960]. Physiological responses to hot environments: an account of work done in Singapore, 1948–1953, at the Royal Naval Tropical Research Unit, with an appendix on preliminary work done at the National Hospital for Nervous Diseases, London. London: H. M. Stationery Office.
Maeda T, Kaneko S, Ohta M, Tanaka K, Sasaki A, Fukushima T [2006]. Risk factors for heatstroke among Japanese forestry workers. J Occup Health 48:223–229.
Makaryus JN, Catanzaro JN, Katona KC [2007]. Exertional rhabdomyolysis and renal failure in patients with sickle cell trait: is it time to change our approach? Hematology 12(4):349–352.
Malchaire J, Kampmann B, Havenith G, Mehnert P, Gebhardt HJ [2000]. Criteria for estimating acceptable exposure times in hot working environments: a review. Int Arch Occup Environ Health 73(4):215–220.
Malchaire J, Piette A, Kampmann B, Mehnert P, Gebhardt H, Havenith G, Den Hartog E, Holmer I, Parsons K, Alfano G, Griefahn B [2001]. Development and validation of the predicted heat strain model. Ann Occup Hyg 45(2):123–135.
Marg K [1983]. Evaporative cooling: heatstress and its effect on worker productivity, quality, and safety. Plant Engineering 37(3):73–74.
Mayo Clinic [2011, September 30]. Urine color: causes. Rochester, MN: Mayo Clinic, http://www.mayoclinic.com/health/urine-color/DS01026/DSECTION=causes.
McArdle WD, Katch FI, Katch VI [1996a]. Exercise at medium and high altitude. In: Exercise physiology: energy, nutrition, and human performance. 4th ed. Baltimore: Williams & Wilkins.
McArdle WD, Katch FI, Katch VI [1996b]. Exercise physiology. 4th ed. Baltimore: Williams & Wilkins.
McArdle WD, McArdle FI, Katch VL [2010a]. Exercise performance and environmental stress. In: McArdle WD, McArdle FI, Katch VL, eds. Exercise physiology: nutrition, energy, and human performance. 7th ed. Philadelphia: Lippincott Williams & Wilkins.
McArdle WD, McArdle FI, Katch VL [2010b]. Exercise physiology. In: McArdle WD, McArdle FI, Katch VL, eds. Exercise physiology: nutrition, energy, and human performance. 7th ed. Philadelphia: Lippincott Williams & Wilkins.
McCullough EA, Arpin EJ, Jones B, Konz SA, Rohles FH [1982]. Heat transfer characteristics of clothing worn in hot industrial environments. ASHRAE, Journal of the American Society of Heating Refrigerating and Air-Conditioning Engineers 88:1077–1094.
McKarns JS, Brief RS [1966]. Nomographs give refined estimate of heat stress index. Heat Pip Air Condit 38:113–116.
McMichael AJ [2013]. Globalization, climate change, and human health. N Engl J Med 368(14):1335–1343.
Medline Plus [2011, September 16]. Urine: abnormal color. https://medlineplus.gov/ency/article/003139.htm.
Mekjavic IB, Eiken O [2006]. Contribution of threshold and nonthermal factors to the regulation of body temperature in humans. J Appl Physiol 100:2065–2072.
Melli G, Chaudhry V, Cornblath DR [2005]. Rhabdomyolysis: an evaluation of 475 hospitalized patients. Medicine (Baltimore) 84(6):377–385.
Meyer F, Bar-Or O, MacDougall D, Heigenhauser GJ [1992]. Sweat electrolyte loss during exercise in the heat: effects of gender and maturation. Med Sci Sports Exerc 24(7):776–781.
Mieusset R, Bujan L, Mansat A, Pontonnier F, Grandjean H [1987]. Effects of artificial cryptorchidism on sperm morphology. Fertil Steril 47(1):150–155.
Minard D [1961]. Prevention of heat casualties in Marine Corps recruits: period of 1955–60, with comparative incidence rates and climatic heat stresses in other training categories. Military Med 126:261–272.
Minard D [1973]. Physiology of heat stress. In: National Institute for Occupational Safety and Health, United States Public Health Service, Division of Occupational Health, eds. The industrial environment: its evaluation & control. 3rd ed. Washington, DC: U.S. Government Printing Office.
Minard D, Copman L [1963]. Elevation of body temperature in disease. In: Hardy JD, ed. Temperature: its measurement and control in science and industry. Vol. 3. New York: Reenhold Co.
Minson CT, Wladkowski SL, Cardell AF, Pawelczyk JA, Kenney WL [1998]. Age alters the cardiovascular response to direct passive heating. J Appl Physiol 84(4):1323–1332.
Montain SJ, Cheuvront SN [2008]. Fluid, electrolyte and carbohydrate requirements for exercise. In: Taylor NAS, Groeller H, eds. Physiological bases for human performance during work and exercise. Edinburgh: Churchhill Livingstone Elsevier.
Montain SJ, Cheuvront SN, Sawka MN [2006]. Exercise associated hyponatraemia: quantitative analysis to understand the aetiology. Br J Sports Med 40(2):98–105; discussion, 198–105.
Moran DS, Erlich T, Epstein Y [2007]. The heat tolerance test: an efficient screening tool for evaluating susceptibility to heat [case report]. J Sport Rehab 16(3):215–221.
Moseley PL [1994]. Mechanisms of heat adaptation: thermotolerance and acclimatization. J Lab Clin Med 123(1):48–52.
MSHA [1976]. U.S. Department of Labor, Mine Safety and Health Administration, National Mine Safety and Health Academy. Heat stress in hot U.S. mines and criteria for standards for mining in hot environments.
MSHA [2001]. U.S. Department of Labor, Mine Safety and Health Administration, National Mine Safety and Health Academy. Heat stress in mining. MSHA safety manual number 6, https://www.osha.gov/dts/osta/otm/otm_iii/otm_iii_4.html.
Mutchler JE, Malzahn DD, Vecchio JL, Soule RD [1976]. An improved method for monitoring heat stress levels in the workplace. American Industrial Hygiene Association Journal 37(3):151–164.
Nag PK, Pradhan CK, Nag A, Ashtekar SP, Desai H [1998]. Efficacy of a water-cooled garment for auxiliary body cooling in heat. Ergonomics 41(2):179–187.
Nauss MD, Schmidt EL, Pancioli AM [2009]. Viral myositis leading to rhabdomyolysis: a case report and literature review. Am J Emerg Med 27(3):372.e5–6.
Navy Environmental Health Center [2007]. Prevention and treatment of heat and cold stress injuries. Technical Manual NEHC-TM-OEM 6260.6A. Portsmouth, VA: Navy Environmental Health Center, Bureau of Medicine and Surgery, http://www.public.navy.mil/surfor/Documents/6260_6A_NMCPHC_TM.pdf.
Nilsson M, Kjellstrom T [2010]. Climate change impacts on working people: how to develop prevention policies. Glob Health Action 3.
NIOSH [1972]. Criteria for a recommended standard: occupational exposure to hot environments. Cincinnati, OH: U.S. Department of Health, Education, and Welfare, Health Services and Mental Health Administration, National Institute for Occupational Safety and Health, DHEW (NIOSH) Publication No. HSM 72-10269.
NIOSH [1985]. Occupational safety and health guidance manual for hazardous waste site activities. Cincinnati, OH: U.S. Department of Health and Human Services, Centers for Disease Control, National Institute for Occupational Safety and Health, DHHS (NIOSH) Publication No. 85-115.
NIOSH [1986a]. Criteria for a recommended standard: occupational exposure to hot environments: revised criteria 1986. Cincinnati, OH: U.S. Department of Health and Human Services, Centers for Disease Control, National Institute for Occupational Safety and Health, DHHS (NIOSH) Publication No. 86-113.
NIOSH [1986b]. Working in hot environments, revised 1986. Cincinnati, OH: U.S. Department of Health and Human Services, Centers for Disease Control, National Institute for Occupational Safety and Health, DHHS (NIOSH) Publication No. 86-112.
NIOSH [1997]. Fire fighter dies of heat stroke while making a fire line during a wildland fire in California. Cincinnati, OH: U.S. Department of Health and Human Services, Centers for Disease Control and Prevention, National Institute for Occupational Safety and Health. Fatality Assessment and Control Evaluation (FACE) Investigation Report No. 97CA01001.
NIOSH [2002]. Landscape mowing assistant dies from heat stroke. Cincinnati, OH: U.S. Department of Health and Human Services, Centers for Disease Control and Prevention, National Institute for Occupational Safety and Health. Fatality Assessment and Control Evaluation (FACE) Investigation Report No. 02-MI-75-01.
NIOSH [2003a]. Assessment of physical hazards at an automobile parts manufacturing facility. Cincinnati, OH: U.S. Department of Health and Human Services, Centers for Disease Control and Prevention, National Institute for Occupational Safety and Health. HETA 2003-0268-3065.
NIOSH [2003b]. Evaluation of heat stress at a glass bottle manufacturer. Cincinnati, OH: U.S. Department of Health and Human Services, Centers for Disease Control and Prevention, National Institute for Occupational Safety and Health. HETA 2003-0311-3052.
NIOSH [2004]. Construction laborer dies from heat stroke at end of workday. Cincinnati, OH: U.S. Department of Health and Human Services, Centers for Disease Control and Prevention, National Institute for Occupational Safety and Health. Fatality Assessment and Control Evaluation (FACE) Investigation Report No. 03KY053.
NIOSH [2006a]. Heat stress and strain evaluation among aluminum potroom employees—Texas. Cincinnati, OH: U.S. Department of Health and Human Services, Centers for Disease Control and Prevention, National Institute for Occupational Safety and Health. HETA 2006-0307-3139.
NIOSH [2006b]. Migrant farm worker dies from heat stroke while working on a tobacco farm: North Carolina. Cincinnati, OH: U.S. Department of Health and Human Services, Centers for Disease Control and Prevention, National Institute for Occupational Safety and Health. Fatality Assessment and Control Evaluation (FACE) Investigation Report No. 2006-04.
NIOSH [2010]. Fire fighter trainee suffers fatal exertional heatstroke during physical fitness training: Texas. Cincinnati, OH: U.S. Department of Health and Human Services, Centers for Disease Control and Prevention, National Institute for Occupational Safety and Health. Fatality Assessment and Control Evaluation (FACE) Report No. 2009-17.
NIOSH [2012]. Wildland fire fighter dies from hyperthermia and exertional heatstroke while conducting mop-up operations: Texas. Cincinnati, OH: U.S. Department of Health and Human Services, Centers for Disease Control and Prevention, National Institute for Occupational Safety and Health. Fatality Assessment and Control Evaluation (FACE) Report No. 2012-17.
NIOSH [2013]. Workplace solutions: preventing heat-related illness or death of outdoor workers. Cincinnati, OH: U.S. Department of Health and Human Services, Centers for Disease Control and Prevention, National Institute for Occupational Safety and Health. Publication No. 2013-143.
NIOSH [2014a]. Evaluation of ergonomic risk factors, thermal exposures, and job stress at an airline catering facility. Cincinnati, OH: U.S. Department of Health and Human Services, Centers for Disease Control and Prevention, National Institute for Occupational Safety and Health. HETA 2011-0131-3221.
NIOSH [2014b]. Evaluation of heat stress, heat strain, and rhabdomyolysis in park employees. Cincinnati, OH: U.S. Department of Health and Human Services, Centers for Disease Control and Prevention, National Institute for Occupational Safety and Health. HETA 2013-0109-3214.
Nishi Y [1981]. Measurement of thermal balance of man. In: Cena K, Clark JA, Politechnika Wrocawska, eds. Bioengineering, thermal physiology, and comfort. Amsterdam: Elsevier Scientific Publishing Co.; New York: Elsevier/North-Holland, distributor.
NOAA [2012]. Heat: a major killer. Silver Spring, MD: National Weather Service, National Oceanic and Atmospheric Administration, http://www.nws.noaa.gov/os/heat/index.shtml.
Nunneley SA [1978]. Physiological responses of women to thermal stress: a review. Med Sci Sports 10(4):250–255.
O’Connor FG, Duester PA [2011]. Rhabdomyolysis. In: Goldman L, Schafer AI, eds. Cecil Medicine. 24th ed. New York: Elsevier Health Sciences.
O’Neal EK, Bishop P [2010]. Effects of work in a hot environment on repeated performances of multiple types of simple mental tasks. Intl J Indust Ergonomics 40(1):77–81.
Olerud JE, Homer LD, Carroll HW [1976]. Incidence of acute exertional rhabdomyolysis: serum myoglobin and enzyme levels as indicators of muscle injury. Arch Intern Med 136(6):692–697.
OSHA [ND]. Monitoring workers at risk of heat-related illness, http://www.osha.gov/SLTC/heatillness/heat_index/monitoring_workers.html. Accessed April 24, 2013.
OSHA [1999]. Heat stress. In: OSHA technical manual, section III, http://www.osha.gov/dts/osta/otm/otm_iii/otm_iii_4.html.
OSHA [2012a]. Heat safety tool, http://www.osha.gov/SLTC/heatillness/heat_index/heat_app.html.
OSHA [2012b]. OSHA’s campaign to prevent heat illness in outdoor workers, https://www.osha.gov/heat/index.html.
OSHA [2012c]. Using the heat index: a guide for employers, http://www.osha.gov/SLTC/heatillness/heat_index/using_heat_protect_workers.html.
OSHA-NIOSH [2011]. OSHA-NIOSH infosheet: protecting workers from heat illness. Cincinnati, OH: U.S. Department of Health and Human Services, Centers for Disease Control and Prevention, National Institute for Occupational Safety and Health. DHHS (NIOSH) Publication No. 2011-174, http://www.cdc.gov/niosh/docs/2011-174/.
Pandolf KB, Burse RL, Goldman RF [1977]. Role of physical fitness in heat acclimatisation, decay and reinduction. Ergonomics 20(4):399–408.
Pandolf KB, Griffin TB, Munro EH, Goldman RF [1980a]. Heat intolerance as a function of percent of body surface involved with miliaria rubra. Am J Physiol 239(3):R233–240.
Pandolf KB, Griffin TB, Munro EH, Goldman RF [1980b]. Persistence of impaired heat tolerance from artificially induced miliaria rubra. Am J Physiol 239(3):R226–232.
Parikh DJ, Pandya CB, Ramanathan NL [1976]. Applicability of the WBGT index of heat stress to work situations in India. Indian J Med Res 64(3):327–335.
Parker RD, Pierce FD [1984]. Comparison of heat stress measuring techniques in a steel mill. Am Ind Hyg Assoc J 45(6):405–415.
Parsons KC [2003]. Human thermal environments: the effects of hot, moderate, and cold environments on human health, comfort, and performance. 2nd ed. London: Taylor and Francis.
Pleet H, Graham JM Jr, Smith DW [1981]. Central nervous system and facial defects associated with maternal hyperthermia at four to 14 weeks’ gestation. Pediatrics 67(6):785–789.
Procope BJ [1965]. Effect of repeated increase of body temperature on human sperm cells [in vitro]. Int J Fertil 10(4):333–339.
Rachootin P, Olsen J [1983]. The risk of infertility and delayed conception associated with exposures in the Danish workplace. J Occup Med 25(5):394–402.
Ramanathan N [1964]. A new weighting system for mean surface temperature of the human body. J Appl Physiol 19(3):531–533.
Ramphal-Naley L [2012]. Screening for heat stress in workers and athletes. Proc (Bayl Univ Med Cent) 25(3):224–228.
Ramsey JD [1975]. Heat stress standard: OSHA’s Advisory Committee recommendations. Natl Safety News 89–95.
Ramsey JD, Beshir MY [2003]. Thermal standards and measurement techniques. In: DiNardi SR, ed. The occupational environment: its evaluation and control. 2nd ed. Cincinnati, OH: American Industrial Hygiene Association.
Ramsey JD, Burford CL, Beshir MY, Jensen RC [1983]. Effects of workplace thermal conditions on safe work behavior. J Safety Res 14:105–114.
Ramsey JD, Morrissey SJ [1978]. Isodecrement curves for task performance in hot environments. Appl Ergon 9(2):66–72.
Rastogi SK, Gupta BN, Husain T [1992]. Wet-bulb globe temperature index: a predictor of physiological strain in hot environments. Occup Med (Lond) 42(2):93–97.
Redmond CK, Emes JJ, Mazumdar S, Magee PC, Kamon E [1979]. Mortality of steelworkers employed in hot jobs. J Environ Pathol Toxicol 2(5):75–96.
Roller WL, Goldman RF [1967]. Estimation of solar radiation environment. Int J Biometeorol 11:329–336.
Rosner MH, Kirven J [2007]. Exercise-associated hyponatremia. Clin J Am Soc Nephrol 2(1):151–161.
Roti MW, Casa DJ, Pumerantz AC, Watson G, Judelson DA, Dias JC, Ruffin K, Armstrong LE [2006]. Thermoregulatory responses to exercise in the heat: chronic caffeine intake has no effect. Aviat Space Environ Med 77(2):124–129.
Rowell LR [1977]. Competition between skin and muscle for blood flow during exercise. In: Nadel ER, American College of Sports Medicine, eds. Problems with temperature regulation during exercise. New York: Academic Press.
Rowell LR [1993]. Human cardiovascular control. New York: Oxford University Press.
Rutstein DD, Mullan RJ, Frazier TM, Halperin WE, Melius JM, Sestito JP [1983]. Sentinel health events (occupational): a basis for physician recognition and public health surveillance. Am J Public Health 73(9):1054–1062.
Sauret JM, Marinides G, Wang GK [2002]. Rhabdomyolysis. Am Fam Phys 65(5):907–912.
Schuckit MA [2011]. Ethanol and methanol. In: Goodman LS, Brunton LL, Gilman A, Chabner B, Knollmann BC, Goodman LS, eds. Goodman & Gilman’s The pharmacological basis of therapeutics. 12th ed. New York: McGraw-Hill Medical.
Schulte PA, Bhattacharya A, Butler CR, Chun HK, Jacklitsch B, Jacobs T, Kiefer M, Lincoln J, Pendergrass S, Shire J, Watson J, Wagner GR [2015]. Advancing the framework for considering the effects of climate change on worker safety and health. Manuscript submitted for publication.
Schulte PA, Chun H [2009]. Climate change and occupational safety and health: establishing a preliminary framework. J Occup Environ Hyg 6(9):542–554.
Semenza JC, Rubin CH, Falter KH, Selanikio JD, Flanders WD, Howe HL, Wilhelm JL [1996]. Heat-related deaths during the July 1995 heat wave in Chicago. N Engl J Med 335(2):84–90.
Serafin WE [1996]. Drugs used in the treatment of asthma. In: Hardman JG, Limbird LE, Molinoff PB, Ruddon RW, Gillman AG, eds. Goodman & Gilman’s The pharmacologic basis of therapeutics. 9th ed. New York: McGraw-Hill.
Shibolet S, Lancaster MC, Danon Y [1976]. Heat stroke: a review. Aviat Space Environ Med 47(3):280–301.
Shvartz E, Benor D [1972]. Heat strain in hot and humid environments. Aerosp Med 43(8):852–855.
Siconolfi SF, Garber CE, Lasater TM, Carleton RA [1985]. A simple, valid step test for estimating maximal oxygen uptake in epidemiologic studies. Am J Epidemiol 121(3):382–390.
Slappendel C, Laird I, Kawachi I, Marshall S, Cryer C [1993]. Factors affecting work-related injury among forestry workers: a review. J Safety Res 24:19–32.
Smith JI, Ramsey JD [1980]. Designing physically demand tasks to minimize levels of worker stress. Industr Engineer 14(44–50).
Spaul WA, Greenleaf JE [1984]. Heat stress field study. US Navy Med 75:25–33.
Springer K [1985]. If you can’t stand the heat. Ohio Monitor 58:4–9.
Stegman J [1981]. Exercise physiology. Chicago: Year Book Medical Publisher.
Stewart JM [1979]. The use of heat transfer and limiting physiological criteria as a basis for setting heat stress limits. Presented at the 2nd International Mine Ventilation Congress, Reno, NV.
Stoecklin-Marois M, Hennessy-Burt T, Mitchell D, Schenker M [2013]. Heat-related illness knowledge and practices among California hired farm workers in The MICASA Study. Ind Health 51(1):47–55.
Strydom NB [1971]. Age as a causal factor in heat stroke. J South Afr Inst Mining Metallurgy 72:112–114.
Strydom NB [1975]. Physical work and heat stress. In: Zenz C, ed. Occupational medicine: principles and practical applications. Chicago: Year Book Medical Publishers.
Strydom NB, Kotze HF, van der Walt WH, Rogers GG [1976]. Effect of ascorbic acid on rate of heat acclimatization. J Appl Physiol 41(2):202–205.
Tanaka M [2007]. Heat stress standard for hot work environments in Japan. Ind Health 45(1):85–90.
Taylor NAS, Kondo N, Kenny WL [2008]. The physiology of acute heat exposure, with implications for human performance in the heat. In: Taylor NAS, Groeller H, eds. Physiological bases of human performance during work and exercise. 1st ed. Edinburgh: Elsevier.
Thacker MT, Lee R, Sabogal RI, Henderson A [2008]. Overview of deaths associated with natural events, United States, 1979–2004. Disasters 32(2):303–315.
Thonneau P, Ducot B, Bujan L, Mieusset R, Spira A [1997]. Effect of male occupational heat exposure on time to pregnancy. Int J Androl 20(5):274–278.
Tillett T [2011]. Pregnancy pause: extreme heat linked to shortened gestation. Environ Health Perspect 119(10):A443.
Tipton M, Pandolf K, Sawka M, Werner J, Taylor N [2008]. Physiological adaptation to hot and cold environments. In: Taylor N, Groeller H, eds. Physiological bases of human performance during work and exercise. Edinburgh: Churchill Livingstone Elsevier.
Tranter M [1998]. An assessment of heat stress among laundry workers in a far North Queensland hotel. J Occup Health Safety Aust N Z 14(1):61–63.
Undem BJ [2006]. Pharmacotherapy of asthma. In: Brunton LL, Lazo JS, Parker KL, eds. Goodman & Gilman’s The pharmacologic basis of therapeutics. 11th ed. New York: McGraw-Hill.
Vaha-Eskeli K, Erkkola R [1991]. The effect of short-term heat stress on uterine contractility, fetal heart rate and fetal movements at late pregnancy. Eur J Obstet Gynecol Reprod Biol 38(1):9–14.
Vogt JJ, Candas V, Libert JP [1982]. Graphical determination of heat tolerance limits. Ergonomics 25(4):285–294.
Vroman NB, Buskirk ER, Hodgson JL [1983]. Cardiac output and skin blood flow in lean and obese individuals during exercise in the heat. J Appl Physiol 55 (1 Pt 1):69–74.
Wallace RF, Kriebel D, Punnett L, Wegman DH, Amoroso PJ [2007]. Prior heat illness hospitalization and risk of early death. Environ Res 104(2):290–295.
Walsh JJ, Page SM [2006]. Rhabdomyolysis and compartment syndrome in military trainees. In: Dekoning B. Recruit Medicine. Washington, DC: Government Printing Office. Washington State Legislature [ND]. WAC 296-62-09510: Scope and purpose, http://apps.leg.wa.gov/WAC/default.aspx?cite=296-62-09510. Accessed March 25, 2013.
Watson S [2011, September 9]. The truth about urine. http://www.webmd.com/urinary-incontinence-oab/features/the-truth-about-urine.
Wells CL, Buskirk ER [1971]. Limb sweating rates overlying active and nonactive muscle tissue. J Appl Physiol 31(6):858–863.
WHO [1969]. Health factors involved in working under conditions of heat stress. Geneva: World Health Organization.
Williams WJ, Coca A, Roberge R, Shepherd A, Powell J, Shaffer RE [2011]. Physiological responses to wearing a prototype fire fighter ensemble compared with a standard ensemble. J Occup Environ Hyg 8:49–57.
Williams WJ, Schneider SM, Stuart CA, Gretebeck RJ, Lane HW, Whitson PA [2003]. Effect of dietary sodium and fluid/electrolyte regulation in humans during bed rest. Aviat Space Environ Med 74(1):37–46.
Wilson E [2008]. Heat stress prevention heats up in California. EHS Today.
Witten L [1980]. Comments on mathematical models for thermoregulatory behavior. Presented at the NIOSH Workshop on Recommended Heat Stress Standards, Cincinnati, OH.
Wrenn KD, Oschner I [1989]. Rhabdomyolysis induced by a caffeine overdose. Ann Emerg Med 18(1):94–97.
Wright HE, Larose J, McLellan TM, Hardcastle SG, Boulay P, Kenny GP [2014]. Moderate-intensity intermittent work in the heat results in similar low-level dehydration in young and older males. J Occup Environ Hyg 11(3):144–153.
Wyndham CH [1973]. The physiology of exercise under heat stress. Annu Rev Physiol 35:193–220.
Wyndham CH [1974]. 1973 Yant memorial lecture: research in the human sciences in the gold mining industry. Am Ind Hyg Assoc J 35(3):113–136.
Wyndham CH, Heyns AJ [1973]. The probability of heat stroke developing at different levels of heat stress. Arch Sci Physiol (Paris) 27(4):545–562.
Xiang J, Bi P, Pisaniello D, Hansen A [2014]. Health impacts of workplace heat exposure: an epidemiological review. Ind Health 52(2):91–101.
Yeargin SW, Casa DJ, Armstrong LE, Watson G, Judelson DA, Psathas E, Sparrow SL [2006]. Heat acclimatization and hydration status of American football players during initial summer workouts. J Strength Cond Res 20(3):463–470.
Zuhl MN, Lanphere KR, Kravitz L, Mermier CM, Schneider S, Dokladny K, Moseley PL [2014]. Effects of oral glutamine supplementation on exercise-induced gastrointestinal permeability and tight junction protein expression. J Appl Physiol 116(2):183–191.
Appendix A
Heat Exchange Equation Utilizing the SI Units
This appendix is referred to in Chapter 3 of the current document. It is reprinted in full from the 1986 NIOSH criteria document:
NIOSH [1986a]. Criteria for a recommended standard: occupational exposure to hot environments: revised criteria 1986. Cincinnati, OH: U.S. Department of Health and Human Services, Centers for Disease Control, National Institute for Occupational Safety and Health.
Convection (C) SI Units
The rate of heat exchange between a person and the ambient air can be stated algebraically:
C = hc(ta−¯tsk)
Where
hc is the mean heat transfer coefficient
ta = air temperature (°C)
¯tsk = air temperature (°C)
The value of hc differs for the different parts of the body [Nishi 1981], depending mainly on the diameter of the body part; for example, at the torso the value of hc is about half of what it is at the thighs. The value used for hc is generally the average of the hc values for the head, chest, back, upper arms, hands, thighs, and legs. The value of hc varies between 2 and 12, depending on body position and activity.
Other factors that influence the value of hc are air speed, air direction, and clothing. The value of ¯tsk can also vary according to the method used for the measurements, the number and location of the measuring points over the body, and the values used for weighting the temperatures measured at the different locations.
Numerous investigations have tried to simplify the calculation of convection heat exchange. The ISO Working Group on the Thermal Environment (ISO-WGTE) developed a draft standard for the Analytical Determination of Heat Stress [ISO 1982b]*. One of the simplifications they adopted is to use only the following three values for hc, which are expressed in units of Wm-2°C-1, corresponding to the SI system.
- When air speed is very low and is due only to natural convection,
hc = 2.38(¯tsk−ta)0.25 - In forced convection when relative air speed (Var) is less than 1m·,
hc = 3.5 + 5.2Var - In forced convection,
when Var is greater than 1m·,
hc = 8.7Var0.6
The expression Var is defined as the ratio of the air velocity relative to the ground and the speed of the body or parts of the body relative to the ground. If the body movement is due to muscular work, Var can be calculated by the following equation:
Var = Va + 0.0052(M−58)
where
Va = air velocity in ms-1
M = metabolic heat production (Wm-2)
For simplicity, however, it is recommended to add 0.7 ms-1 to Va as a correction for the effect of physical work.
The ISO-WGTE recommends also to include in the equation for calculating the convection heat exchange a separate coefficient for clothing, called a reduction factor for loss of sensible heat exchange due to the wearing of clothes (Fcl), which can be calculated by the following equation:
Fcl = 1/1 + (hc + hr)Icl (dimensionless)
where
hr = the heat transfer coefficient for radiant heat exchange
Icl = the thermal insulation of clothing
Both hr and Icl will be explained later in this appendix in more detail. The ISO-WGTE recommended the use of 36°C (96.8°F) for tsk on the assumption that most workers engaged in hot jobs would have a tsk very close to this temperature; thus, any error resulting from this simplification will be small. They also assumed that most corrected for different body positions when calculating the convective heat exchange of workers.
The final equation for C to be used according to the ISO-WGTE is
C = hcFcl (ta−36) (Wm-2)
Radiation (R) SI Units
The rate of radiant heat exchange between a person and the surrounding solid objects can be stated algebraically:
R = hr (Tr−Tsk)4
where
hr = the coefficient for radiant heat exchange
Tr = the mean radiant temperature in °K
Tsk = the mean weighted skin temperature in °K
The value of hr depends on the body position of the exposed worker and on the emissivity of the skin and clothing, as well as on the insulation of clothing. The body position will determine how much of the total body surface will be actually exposed to radiation, and the emissivity of the skin and clothing will determine how much of the radiant heat energy will be absorbed on those surfaces. The insulation of clothing determines how much of the radiant heat absorbed at the surface of the garments will actually be transferred to the skin.
The ISO-WGTE recommended a linearized equation for calculating the value of R, using SI units:
R = hr Fcl (tr − tsk) (Wm-2 / °C-1)
The effect of insulation and emissivity of the clothing material on radiant heat exchange is covered by the addition of the clothing coefficient Fcl, which is also used in the equation for C as described above.
They also recommend a simplified equation for calculating an approximate value for hr:
hr = 4Esk Ar /ADu [(tr + tsk)/2 + 273]3
= the universal radiation constant
= (5.67 × 10-8) Wm-2 °K-4
The effect of the emissivity of the skin on radiant heat exchange is covered by the expression Esk, which has the value of 0.97 in the infrared range. The effect of body position is covered by the expression Ar/ADu, which is the ratio of the skin surface area exposed to radiation and the total skin surface area, as estimated by DeBois’ formula:
ADu = 0.00718 × Weight0.425 / Height0.725
In this equation, body weight must be expressed in kg and height in cm, and the value of ADu is then obtained in m2. Values given for the ratio Ar/ADu by the ISO-WGTE include these:
Standing: 0.77
Seated: 0.70
Crouched: 0.67
The value of tr (mean radiant temperature) can be calculated by the following equation:
tr = tg + 1.8Va0.5(tg – ta)
For further simplification, the value of tsk can be assumed to be 36°C, just as it was in the equation for convection.
Evaporation (E) SI Units
Ereq is the amount of heat that must be eliminated from the body by evaporation of sweat from the skin in order to maintain thermal equilibrium. However, there are major limitations to the maximum amount of sweat that can be evaporated from the skin (Emax):
- The human sweating capacity
- The maximum vapor uptake capacity of the ambient air
- The resistance of the clothing to evaporation
As described in Chapter 5†, the sweating capacity of healthy individuals is influenced by age, sex, state of hydration, and acclimatization.
The draft ISO-WGTE [ISO 1982b]‡ standard recommends that an hourly sweat rate of 650 grams for an unacclimatized person and 1,040 grams for an acclimatized person are the maximum permissible for the average worker while performing physical work in heat. However, these sweating rate limits are not the maximum sweating capacities; they are related to levels of heat stress at which the risk of heat-related illnesses is minimal.
For a full work shift, the total sweat output should not exceed 3,250 grams for an unacclimatized person and 5,200 grams for an acclimatized person to prevent deterioration in performance due to dehydration. It follows from the foregoing that if heat exposure is evenly distributed over an 8-hour shift, then the maximum acceptable hourly sweat rate is about 400 grams for an unacclimatized person and 650 grams for an acclimatized person.
Thus, if the worker’s heat exposure remains within the limits of the recommended standard, then the maximum sweating capacity will not be exceeded, and the limitation of evaporation will be due only to the maximum vapor uptake capacity of the ambient air. The Emax can be described with the equation recommended by the ISO-WGTE:
Emax = (psk,s-pa)/Re
where
Emax = maximum water vapor uptake capacity (Wm-2)
psk,s = saturated water vapor pressure at 36°C skin temperature (5.9 kPa)
pa = partial water vapor pressure at ambient air temperature (kPa)
Re = total evaporative resistance of the limiting layer of air and clothing (m2kPa W-1), which can be calculated by the following equation:
Re = 1 / 16.7 / hc / Fpcl
where
hc = convective heat exchange coefficient (Wm-2 / C-1)
Fpcl = reduction factor for loss in latent heat exchange due to clothing (dimensionless) which
can be calculated by the following equation:
Fpcl = 1 / 1 + 0.92hc / Icl
where
Icl = Thermal insulation of clothing (m2 °C W-1)
What this means is that the maximum vapor uptake capacity of the air depends on the temperature, humidity, and velocity of the ambient air and clothing worn. However, the relationship of these variables with respect to human heat tolerance is quite complex. Further complications are caused by the fact that in order to be able to evaporate a certain amount of sweat from the skin, it is necessary to sweat more than that amount, because some of the sweat will drip off the skin or will be picked up by the clothing. To calculate the additional amount of sweat required due to dripping, the ISO-WGTE recommended the following equations:
Sreq = Ereq
where
Sreq = required sweat (Wm-2). This quantity can also be expressed as (g h-1 m-2) × 0.68
Ereq = required evaporation (Wm-2), which can be calculated by the equation Ereq = M+C+R
η = Evaporative efficiency of sweating of a nude person, which can be calculated by the following equation:
η = 1 – 0.5 / e-6.6(1-w)
where
e = the base of natural logarithm
w = Ereq/Emax, also called the “wettedness index”
The experimental data are insufficient to calculate the loss of evaporative efficiency of sweat due to the wicking effect of clothing. However, if the workers wear thin knitted cotton underwear, this can actually enhance the cooling efficiency of sweat, because after wicking the sweat off the skin, it spreads it more evenly over a larger area, thus enhancing evaporation and preventing dripping. Since the thin knitted material clings to the skin, the evaporative cooling will affect the skin without much loss to the environment. If a loosely fitting garment wicks up the sweat, there may be a substantial loss in evaporative cooling efficiency. However, if the heat exposure (M+C+R) remains below the human sweating capacity, then the exposed worker’s sweat excretion can increase to compensate for the loss of its cooling efficiency. A compensatory increase of sweating does not add much to the physiologic strain if water and electrolytes are replaced satisfactorily and if the water vapor uptake capacity of the ambient air is not exhausted.
In order to make sure that in the Sreq index the wettedness modifies the value of Sreq only to the extent to which it increases physiologic strain, the Ereq/Emax ratio affects the value of Sreq in an exponential manner.
The closer the value of Ereq comes to Emax, the greater the impact of w on Sreq. This is in accord with the physiologic strain as well as the subjective feeling of discomfort.
In this manner, the Sreq index is an improvement over other rational heat stress indices, but at the same time the calculations involved are more complex. With the availability of pocket-sized programmable calculators, the problem of calculations required is greatly reduced. However, it is questionable whether it is worthwhile to perform a complex calculation with variables that cannot be measured accurately. These variables include the mean weighted skin temperature, the velocity and direction of the air, the body position and exposed surface area, the insulation and vapor permeability of the clothing, and the metabolic heat generated by the work.
For practical purposes, simplicity of the calculations may be preferable to all-inclusiveness. Also, the utilization of familiar units such as kcal, Btu, and W, (the British units or metric units instead of the SI suggested), to express energy in heat production may increase the use of the calculations. They can be useful in analysis of a hot job for determining the optimal method of stress reduction and for prediction of the magnitude of heat stress so that proper preventive work practices and engineering controls can be planned in advance.
Footnotes for Appendix A
*This standard has been revised. See Ergonomics of the Thermal Environment: Analytical Determination and Interpretation of Heat Stress Using Calculation of the Predicted Heat Strain (Standard No. ISO 7933) [ISO 2004b].
†Chapter 4 of the current document.
‡This standard has been revised. See Ergonomics of the Thermal Environment: Analytical Determination and Interpretation of Heat Stress Using Calculation of the Predicted Heat Strain (Standard No. ISO 7933) [ISO 2004b].
Appendix B
Urine Chart
Urine charts can be implemented as a training tool to demonstrate the concept of color change between the urine of a well-hydrated worker and that of a dehydrated worker. When conducting an investigation to evaluate the validity and sensitivity of urine color, Armstrong et al. [1998] found that urine color was as good an index as urine osmolality, urine specific gravity, urine volume, plasma osmolality, plasma sodium, and plasma total protein for tracking changes in body water and hydration status. In an earlier study, the author suggested that urine color could be used in workplace settings where close estimates of urine specific gravity or urine osmolality are acceptable [Armstrong et al. 1994, 2010].
Although the urine chart is a good indicator of hydration status for most workers with normal pale yellow to deep amber urine, urine color can also be affected by diet, medications, and illnesses or disorders. See Table B-1.
Appendix C
Heat Index
Adapted from NOAA [2012].
The National Oceanic and Atmospheric Administration (NOAA) issues heat alerts based on the heat index values, as seen in the chart above. The Heat Index is a measure of how hot it feels when RH is taken into account with the actual air temperature. Since heat index values were devised for shady, light wind conditions, exposure to full sunshine can increase heat index values by up to 15°F.
NOAA may also issue an extreme heat advisory:
- Excessive Heat Outlook
Extended excessive heat (heat index of 105°F−110°F [41°C−43°C]) over the next 3 to 7 days. - Excessive Heat Watch
Excessive heat may occur within the next 24 to 72 hours. - Excessive Heat Warning
The heat index will be life threatening in the next 24 hours. Excessive heat is imminent or has a high probability of occurring. - Excessive Heat Advisory
The heat index may be uncomfortable but not life threatening if precautions are taken.
NOAA uses four bands of colors associated with four risk levels. The risk level–related measures in Table C-1 have been modified by OSHA for use on worksites.
Disclaimer
Mention of any company or product does not constitute endorsement by the National Institute for Occupational Safety and Health (NIOSH). In addition, citations of websites external to NIOSH do not constitute NIOSH endorsement of the sponsoring organizations or their programs or products. Furthermore, NIOSH is not responsible for the content of these websites.
Suggested Citation
NIOSH [2016]. NIOSH criteria for a recommended standard: occupational exposure to heat and hot environments. By Jacklitsch B, Williams WJ, Musolin K, Coca A, Kim J-H, Turner N. Cincinnati, OH: U.S. Department of Health and Human Services, Centers for Disease Control and Prevention, National Institute for Occupational Safety and Health, DHHS (NIOSH) Publication 2016-106.
Ordering Information
This document is in the public domain and may be freely copied or reprinted. To receive NIOSH documents or other information about occupational safety and health topics, contact NIOSH at
Telephone: 1-800-CDC-INFO (1-800-232-4636)
TTY: 1-888-232-6348
E-mail: cdcinfo@cdc.gov
or visit the NIOSH website at www.cdc.gov/niosh. For a monthly update on news at NIOSH, subscribe to NIOSH eNews by visiting www.cdc.gov/niosh/eNews.
February 2016
DEPARTMENT OF HEALTH AND HUMAN SERVICES
Centers for Disease Control and Prevention
National Institute for Occupational Safety and Health